FDRC Seminar Series
Each semester, FDRC invites speakers from across the United States and abroad to present their research on fluid dynamics to members of the Penn State community. Topics include fundamental research on turbulence, numerical methods for CFD, the development of experimental techniques, and engineering applications related to medicine, propulsion, combustion, and more.
Seminars are accompanied by complimentary coffee and donuts!
Fall 2024 Series
Seminars in this series are hosted every Thursday at 9:30 am in 125 Reber Building.
Schedule
Date | Speaker | Affiliation | Host |
---|---|---|---|
Aug. 29 | Sebanti Chattopadhyay | Pennsylvania State University | Nathan Kein |
Sep. 5 | Frank Engel | United States Geological Survey | Roberto Fernández |
Sep. 12 | Melissa Brindise | Pennsylvania State University | Robert Kunz |
Sep. 19 | Hiroaki Nishikawa | National Institute of Aerospace | James Coder |
Sep. 26 | Ralph Volino | United States Naval Academy | Xiang Yang |
Oct. 3 | Justin Jaworski | Virginia Tech | Zachary Berger |
Oct. 10 | Paul Milewski | Pennsylvania State University | Tamy Guimarães |
Oct. 17 | Karthik Duraisamy | University of Michigan | Xiang Yang |
Oct. 24 | Brett Bornhoft | Air Force Research Lab | Xiang Yang |
Oct. 31 | Binbin Wang | University of Missouri | Xiaofeng Liu |
Nov. 7 | James Goldschmidt | Penn State Applied Research Laboratory | Zachary Berger |
Nov. 14 | Christopher Douglas | Duke University | Samuel Grauer |
Nov. 21 | Gary Settles | Pennsylvania State University | Samuel Grauer |
Nov. 28 | Thanksgiving | ||
Dec. 5 | Sayantan Bhattacharya | University of Maryland, Baltimore County | Melissa Brindise |
Dec. 12 | Pedro Sáenz | University of North Carolina | Paul Milewski |
Abstracts and Biosketches
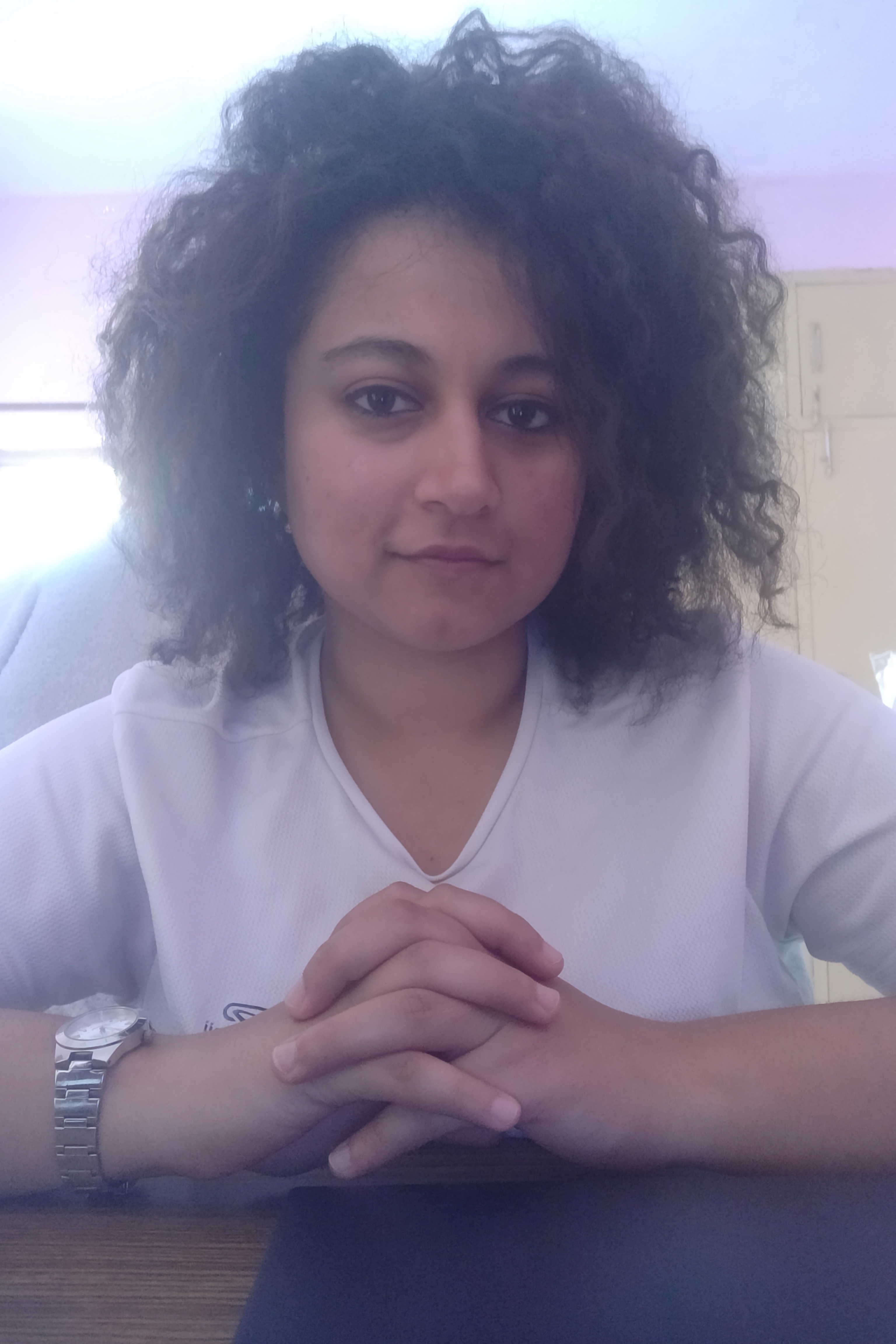
Sebanti Chattopadhyay
Postdoctoral Scholar, Department of Physics
Pennsylvania State University
Yielding and memory formation in an adhesive granular gel
August 29, 2024
Dense granular suspensions are an important class of soft materials that have drawn significant research attention in recent years, particularly for their huge application-potential in designing smart and adaptive materials. In this work, we study yielding of dense suspensions of adhesive granular particles using rheology and in-situ imaging. We correlate the yielding behaviour with the inter-particle rearrangements and shear banding in the system. We propose a generalized yielding phase diagram that depends on various critical jamming volume fractions measured directly from the particle settling experiments. Finally, we show an interesting memory forming capability in this system under repeated cyclic driving. Remarkably, the encoded memory has a clear signature in the bulk mechanical properties of the system. By using surfactant, we systematically explore the role of inter-particle adhesion in the yielding as well as memory formation in the system. Thus, we demonstrate the ability to tune the system's shear modulus both by external driving as well as by changing the inter-particle interactions.
Biosketch
Dr. Sebanti Chattopadhyay is a postdoctoral scholar in the Department of Physics at the Pennsylvania State University. She did her M.Sc. in physics at the Indian Institute of Technology (IIT) Roorkee, India and subsequently obtained her Ph.D. in physics from Raman Research Institute Bangalore, India in 2024. As part of her Ph.D., she studied the rheology of complex fluids formed by dense particulate suspensions. Currently, she is studying mechanical memory formation in various soft matter and adjacent systems in the lab of Dr. Nathan Keim.
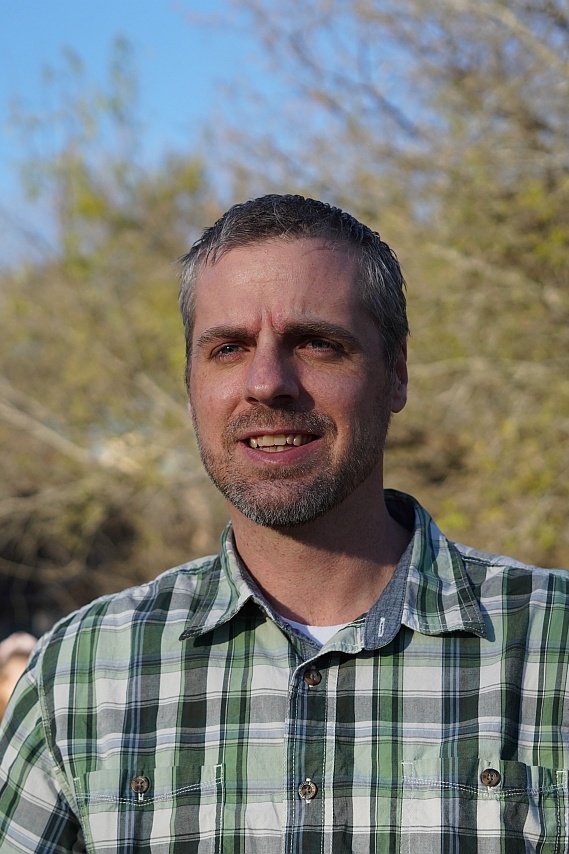
Frank Engel
Geographer, Water Resources Mission Area
United States Geological Survey
Development of image velocimetry software for hydrography: bridging research and applied applications
September 5, 2024
As hydrometric techniques evolve, the operationalization of non-contact methods such as image velocimetry has become increasingly important to the U.S. Geological Survey (USGS), which maintains over 8,500 real-time river monitoring stations across the USA. With the growing need to enhance safety and reduce costs, USGS is working to implement these innovative techniques. The development of generalized image velocimetry software for hydrography marks a significant advancement in translating hydrometric techniques from research into operational practice.
This seminar will discuss the creation and implementation of the Image Velocimetry (IVy) software application that bridges the gap between academic research and applied hydrographic practices. Specifically, IVy software is being used to operationalize Space–Time Image Velocimetry (STIV) methods to determine accurate and reliable streamflow measurements at USGS streamgages.
IVy software addresses the dual challenges USGS and other hydrometric agencies face in ensuring the repeatability of results while reducing the technical expertise required to operate equipment and software. By incorporating tools that standardize workflows and facilitate the review and approval of new measurement methods, IVy ensures consistent, high-quality data production accessible to a wide range of user proficiencies.
This seminar will also explore how IVy has informed the development of policy and procedural documentation necessary for the broader adoption of image velocimetry techniques within hydrometric agencies. These efforts are crucial for ensuring that image velocimetry is not only a research tool but also a robust, operational method for streamflow measurement. The integration of IVy into hydrometric practices represents a significant step forward in improving water resource management, hazard mitigation, and ecological assessments, highlighting the importance of software that can adapt research innovations to practical, everyday applications in the field.
Biosketch
Dr. Frank Engel is a Geographer with the U.S. Geological Survey (USGS), where he specializes in transforming cutting-edge research into operational science. Originally trained as a fluvial geomorphologist, Dr. Engel now focuses on pioneering non-contact measurement techniques for riverine parameters, with the goal of integrating these innovations into the USGS's daily gaging operations. He also leads the development of enterprise-level cyberinfrastructure for cameras and multispectral sensors at gaging stations across the USGS network. Dr. Engel resides in San Antonio, TX, with his wife and two children. He enjoys gravel biking, hiking, and exploring the outdoors.
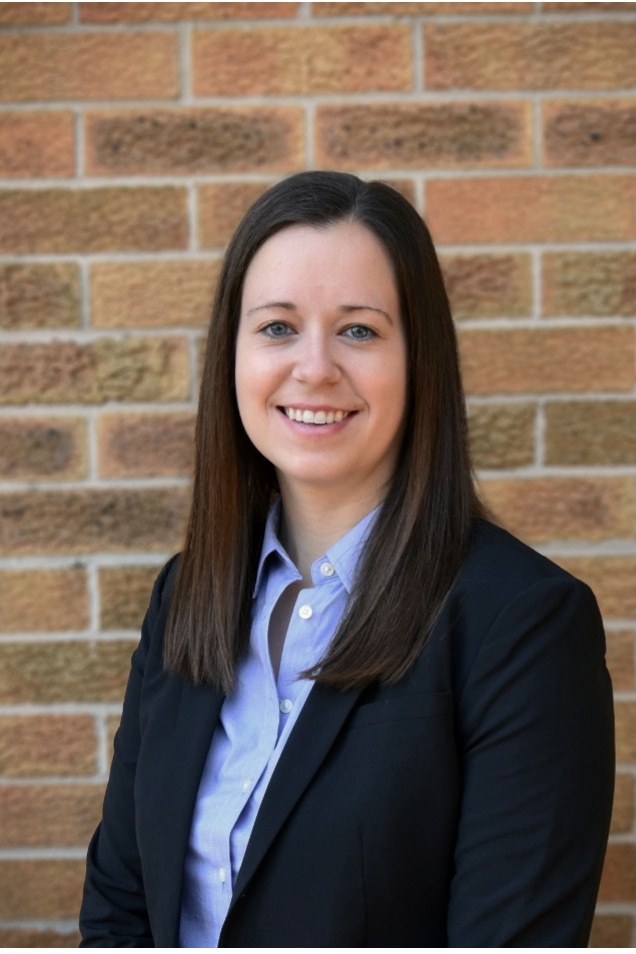
Melissa Brindise
Assistant Professor, Department of Mechanical Engineering
Pennsylvania State University
Characterizing transition to turbulence in pulsatile flows
September 12, 2024
Transition to turbulence in pulsatile flow has been observed across a variety of application areas including biomedical, environmental, and turbomachinery flows. Prior studies, in the biomedical domain for example, have (controversially) reported transitional flow behaviors to exist even in flows with mean Reynolds numbers as low as 250. The intermittent flow structures associated with the transitional regime cause significant fluctuations in flow parameters such as wall shear stress (WSS) and pressure. Quantifying these effects is important as these intermittent flow behaviors can weaken materials to failure, cause performance reductions, and lead to other adversarial effects. However, the mechanisms driving the onset and development of transitional flow remain poorly understood. In this talk, I will discuss the results of our experimental studies which parametrically evaluate how axial flow factors (e.g., changes to Womersley number, inflow waveform shape) affect the onset of transitional flow. Additionally, I will discuss our ongoing efforts to develop a universal metric to characterize the extent to which turbulence-scales exist in any arbitrary flow.
Biosketch
Dr. Melissa Brindise is an Assistant Professor in the Department of Mechanical Engineering at Penn State University. She received her B.S. in Aeronautical Engineering (2013) and Ph.D. in Mechanical Engineering (2019) both from Purdue University. Prior to joining Penn State in 2021, she was a post-doctoral research associate at Purdue as part of the Eli Lilly–Purdue partnership. Her research combines experimental flow physics with image and signal processing to improve analysis methods and current clinical understanding of neuro- and cardiovascular disease, injury, function, and treatment. Broadly, her lab focuses on 1) advancing our understanding of the role of hemodynamics and vascular changes in the onset, progression, and efficacy of treatment of diseases and 2) developing objective, evidence-based methods to transform how we interpret patient data. Her current research interests include the application areas of cerebral aneurysms, Moyamoya disease, cognitive function, heart disease and arrhythmias, and transition to turbulence in unsteady flows.
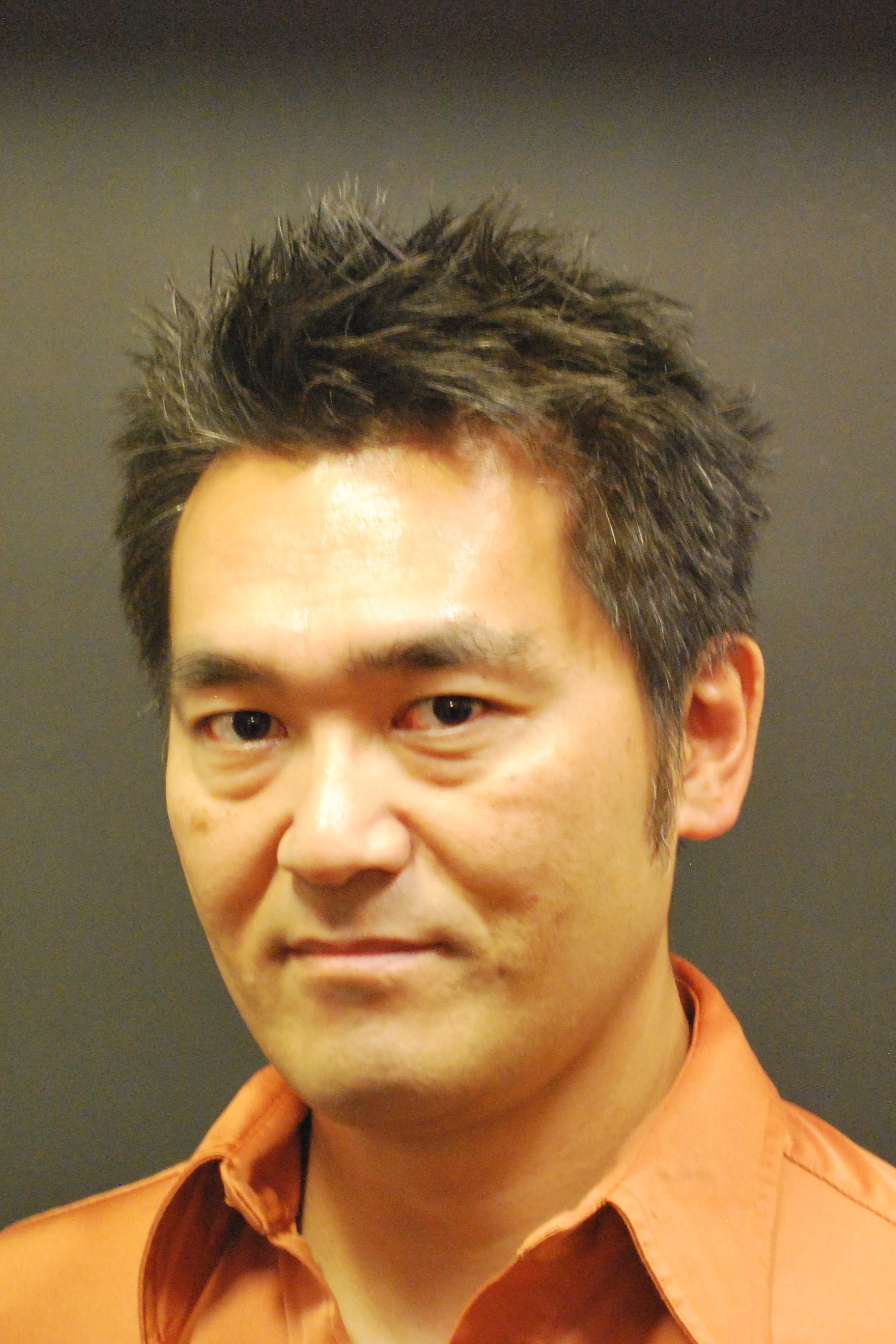
Hiroaki Nishikawa
Senior Research Fellow, Center for High Performance Aerodynamic Computing
National Institute of Aerospace
A hyperbolic Navier–Stokes method for
efficient viscous discretizations and accurate gradients on irregular grids
September 19, 2024
In this seminar, I will talk about an idea of numerically solving, ultimately, all partial-differential equations as hyperbolic systems. Hyperbolic formulations will be presented for diffusion and Navier–Stokes equations. Then, these systems can be easily discretized by methods for hyperbolic systems just like advection and inviscid equations. The resulting solver can be orders-of-magnitude faster than conventional diffusion/viscous solvers. Moreover, it can produce highly smooth and accurate gradients (e.g., diffusive fluxes, vorticity, viscous stresses, and heat fluxes) on highly irregular grids, which is essential to successful automated CFD simulations with fully adaptive grids. I will explain how such solvers can be constructed via hyperbolization, present numerical results, and discuss current and future developments.
Biosketch
Dr. Hiro Nishikawa is a research fellow at National Institute of Aerospace (NIA). He earned Ph.D. in Aerospace Engineering and Scientific Computing at University of Michigan in 2001, and he then worked as a postdoctoral fellow at the University of Michigan on adaptive grid methods, local preconditioning methods, semi-coarsening multigrid methods, rotated-hybrid Riemann solvers, and high-order upwind/viscous schemes. He joined NIA in 2007 and has been working on CFD algorithm research at NASA Langley Research Center, focusing on the hyperbolic method, economical third-order discretization methods, and other improved algorithms for practical unstructured-grid CFD solvers.
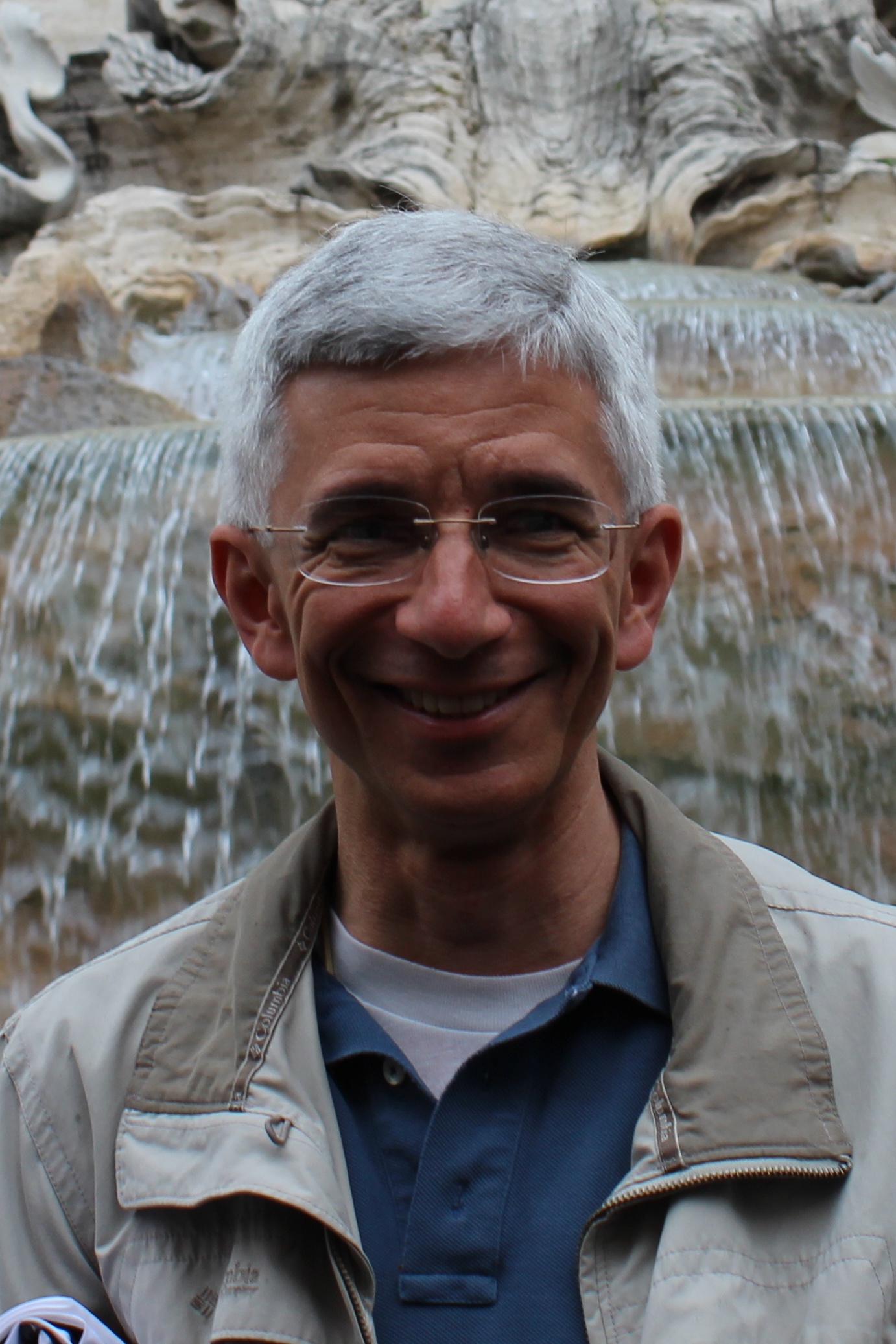
Ralph Volino
Professor, Department of Mechanical and Nuclear Engineering
United States Naval Academy
Effects of roughness on turbulent boundary layers under non-zero pressure gradient conditions
September 26, 2024
Surface roughness has a significant effect on boundary layer behavior in many flows of practical interest, including naturally occurring flows such as those in the atmospheric boundary layer and flows of engineering interest such as those over naval vessels and aircraft or within turbomachinery. Roughness typically increases drag, can induce transition from laminar to turbulent flow, and in adverse pressure gradients (APG) increases the likelihood of boundary layer separation. Prediction of flows over rough surfaces is, therefore, of critical importance. The most studied and well understood boundary layer, in contrast, is the canonical, zero pressure gradient (ZPG) boundary layer on a smooth flat plate. Most turbulence models are based on the canonical case, with the assumption that roughness does not change the turbulence structure in the outer part of the boundary layer, and roughness effects can be captured through the boundary condition at the wall. For the ZPG case, there is considerable evidence that supports the assumption of outer layer similarity between rough- and smooth-wall cases. For non-ZPG flows, the validity of the assumption and the conditions needed for similarity are less clear. This seminar will present current experimental work that documents the flow over smooth and rough surfaces for a range of favorable (FPG) and adverse pressure gradient conditions. The results indicate that similarity largely holds in FPG cases unless the FPG is strong enough to induce relaminarization, but the APG case is considerably more complicated.
Biosketch
Dr. Ralph Volino is a Professor in the Department of Mechanical and Nuclear Engineering at the United States Naval Academy. He received his B.S. in Mechanical Engineering from Michigan State University, and his M.S. and Ph.D. in Mechanical Engineering from the University of Minnesota. He has held visiting summer positions at the NASA Glenn Research Center, Naval Research Laboratory, and the Air Force Research Laboratory. He studies the fluid mechanics and heat transfer in turbulent and transitional boundary layers. Current efforts are directed at the combined effects of roughness and pressure gradients, with a particular focus on boundary layers approaching separation, and is funded by the Office of Naval Research. His prior work focused mainly on turbomachinery applications, including boundary layer separation and transition on low pressure turbine airfoils, tip leakage flows, and film cooling under unsteady conditions. He is a Fellow of the ASME.
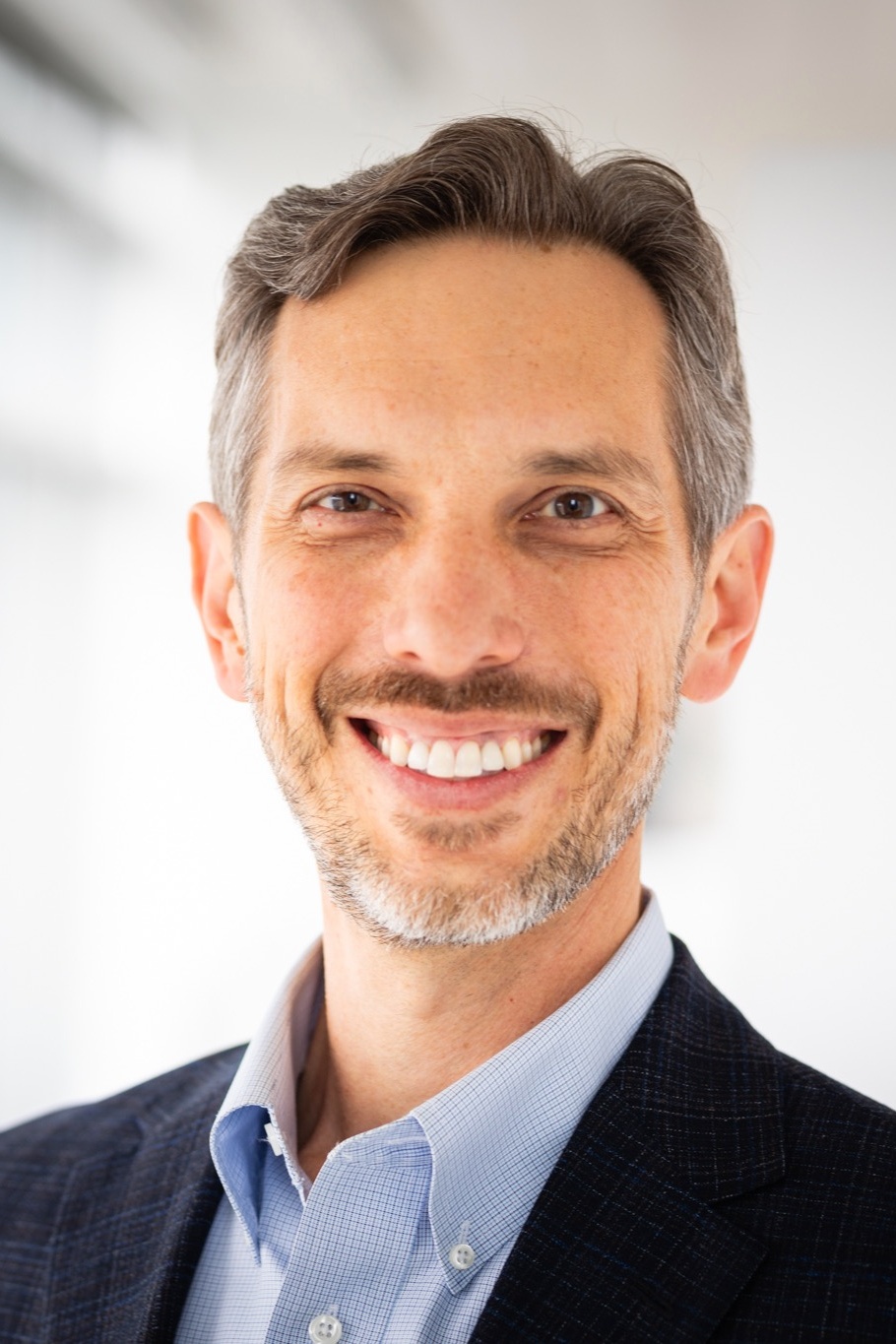
Justin Jaworski
Associate Professor, Kevin T. Crofton Department of Aerospace and Ocean Engineering
Virginia Tech
Aeroacoustics and aerodynamics of quiet owl flight
October 3, 2024
Many owl species rely on specialized plumage to mitigate their aerodynamic noise and achieve functionally-silent flight while hunting. One such plumage feature, a tattered arrangement of flexible trailing-edge feathers, is idealized as a semi-infinite poroelastic plate to model the effects that edge compliance and flow seepage have on the noise production. The associated acoustic scattering problem is solved to identify how the noise scales with the flight velocity, where special attention is paid to the limiting cases of rigid-porous and elastic-impermeable plate conditions. Results from this analysis identify parameter spaces where the porous and/or elastic properties of a trailing edge may be tailored to diminish or effectively eliminate the edge scattering effect, for which experimental validation efforts have been carried out at ARL Penn State. In complement to the acoustic analysis, steady and unsteady aerodynamic models including wing porosity are formulated and solved in anticipation of the potential trade-off of aerodynamic performance and acoustic stealth. The aerodynamic analysis yields porous extensions of the classical unsteady aerodynamic functions and further indicates how porosity may be used as a passive gust rejection strategy.
Biosketch
Dr. Justin Jaworski received his B.S.E., M.S., and Ph.D. (2009) degrees in mechanical engineering from Duke University, where he was awarded the 2008 Dean's Award for Excellence in Mentoring and supported the research of several undergraduate students with competitive grant awards such as the North Carolina Space Grant and Sigma Xi Grant-in-Aid. Before joining the Aerospace and Ocean Engineering faculty at Virginia Tech, he was on the Mechanical Engineering and Mechanics faculty at Lehigh University for 10 years and held the postdoctoral appointments of NRC Research Associate at the Air Force Research Laboratory and NSF International Research Fellow at the University of Cambridge. He is an AIAA Associate Fellow and is a recipient of an AFOSR Young Investigator Award (2015) and an NSF CAREER Award (2019). His present research efforts include the unsteady aeroelastic analyses of flexible structures for next-generation aerospace and energy applications, the vortex dynamics of formation flight, and the development of mathematical models to figure out how owls fly so quietly.
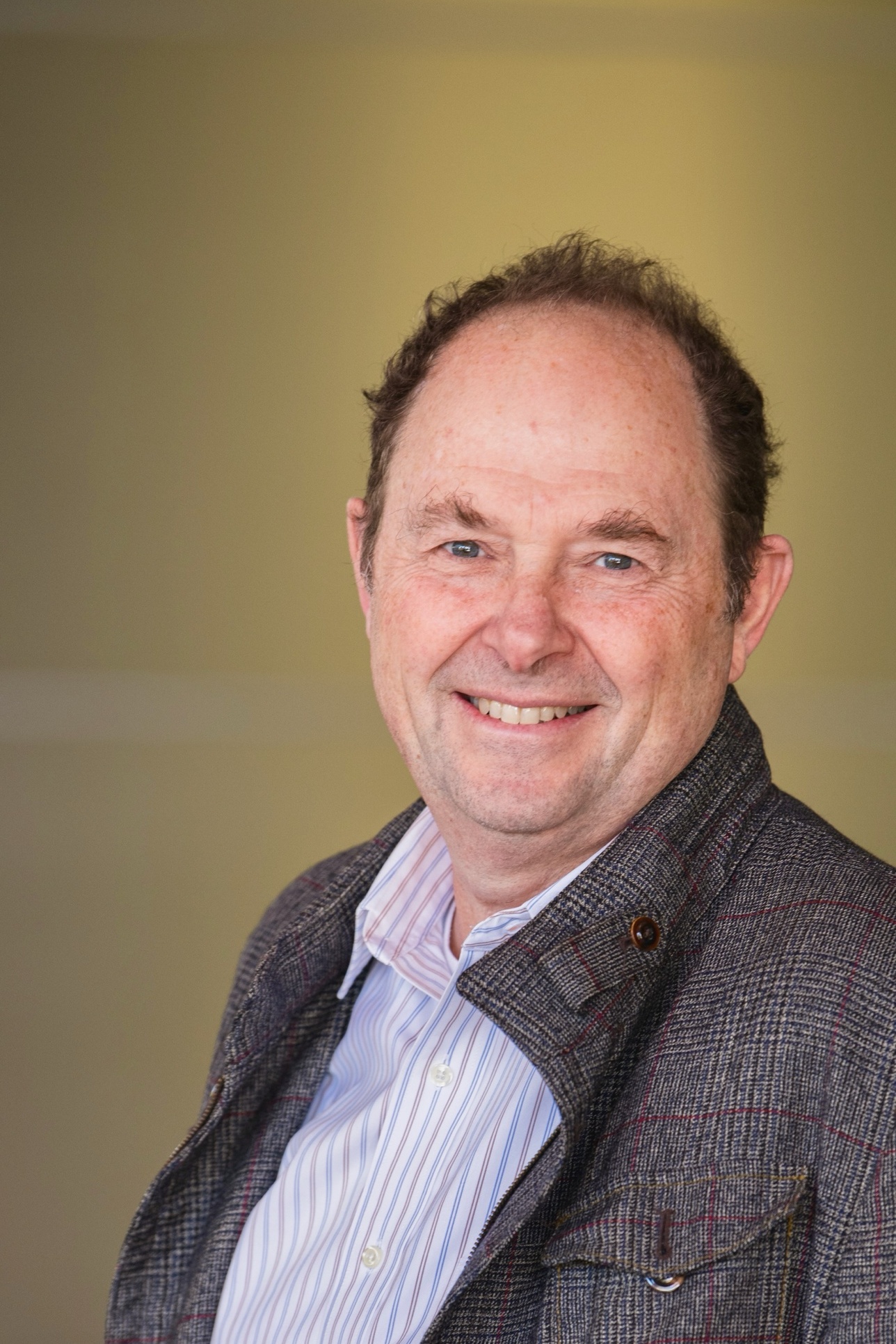
Paul Milewski
Professor, Department of Mathematics
Pennsylvania State University
Resonance of free surface water waves in cylindrical containers
October 10, 2024
Waves sloshing in a container of rectangular cross-section can behave very differently than those in a circular cylinder. This talk covers one aspect of how geometry affects the nonlinear evolution of waves. Nonlinear resonance is a mechanism by which energy is continuously exchanged between a small number of wave modes, and is common to many nonlinear dispersive wave systems. In the context of free-surface gravity waves such as ocean surface waves, nonlinear resonances have been studied extensively over the past 60 years (and were mentioned in the award of the 2021 Nobel Prize in Physics to Klaus Hasselmann) almost always on domains that are large compared to the characteristic wavelength. In this case, the dispersion relation dictates that only quartic (4-wave) resonances may occur. In contrast, nonlinear resonances in confined three-dimensional geometries have received relatively little attention, where, perhaps surprisingly, stronger 3-wave resonances do occur. We will present the results characterizing the configuration and dynamics of resonant triads in cylindrical basins of arbitrary cross sections, demonstrating that these triads are ubiquitous, with a rectangular cross section being an exception where they do not occur.
Biosketch
Dr. Paul Milewski is a Professor and current Department Head in the Department of Mathematics at Penn State. He received his B.Sc. and M.Sc. in Aerospace Engineering at Boston University and his Ph.D. (1993) from Mathematics at M.I.T. Prior to joining Mathematics at Penn State in 2023, he had positions in the Departments of Mathematical Sciences at the University of Bath (UK, 2011–2023), and in Mathematics at Wisconsin–Madison (1995–2011) and Stanford. He has held visiting positions, among others at IMPA (Brazil) and ENS (France). He was recipient of a Royal Society Wolfson Fellowship and a Sloan Fellowship. His research is in applied and computational mathematics, mainly in nonlinear waves in fluids, but also with interests across mathematical modeling of physical and biological systems, and data science.
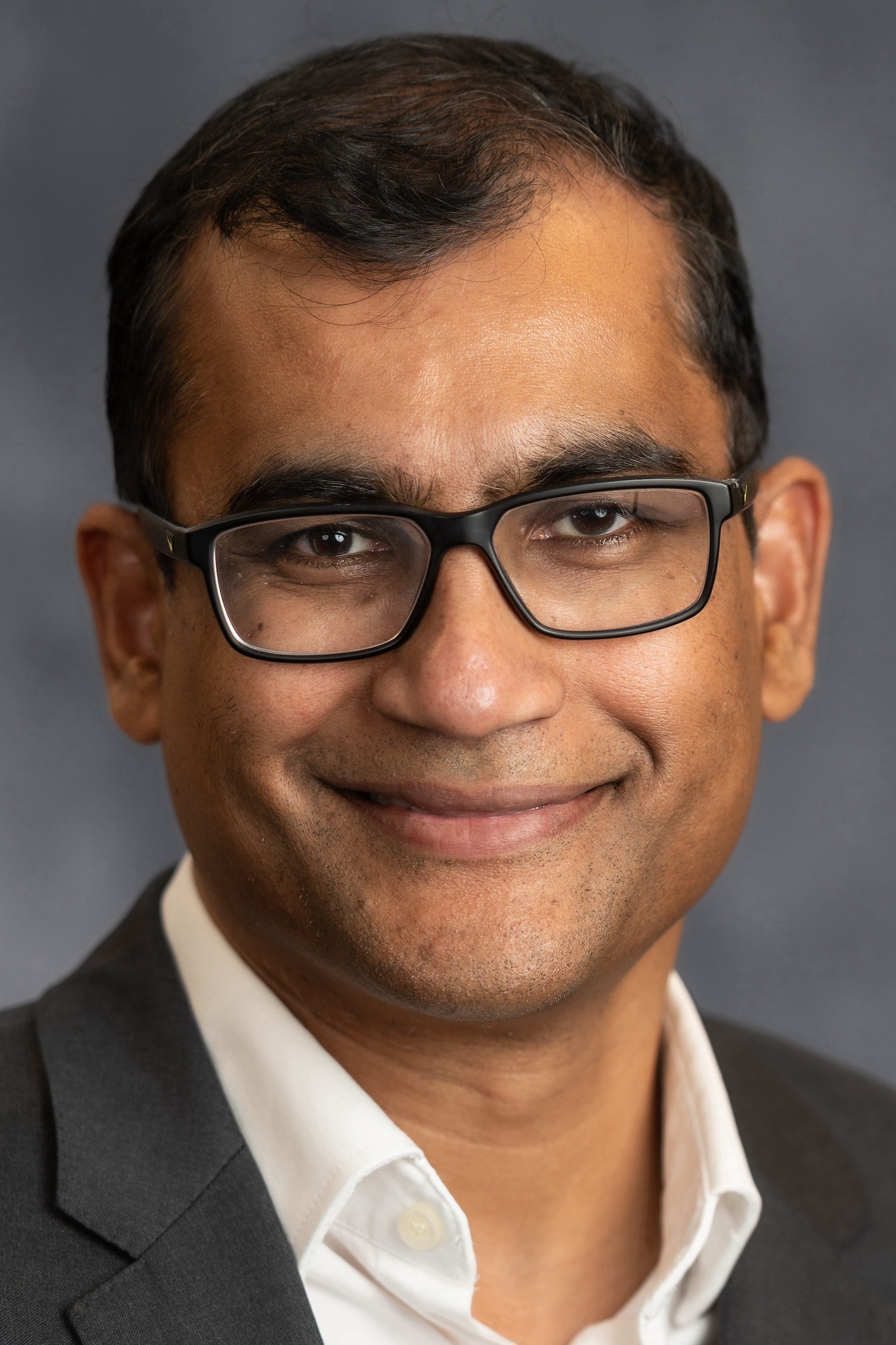
Karthik Duraisamy
Professor, Department of Aerospace Engineering
University of Michigan
Generalizable data-driven predictive modeling of complex physical systems
October 17, 2024
This talk covers two different approaches that are targeted towards the goal of developing generalizable data-driven models for complex physics.
The first part of the talk will present advances towards the development of effective projection-based reduced order models (ROMs) for complex multi-scale problems. As a representative application, we consider combustion dynamics in a rocket engine, which is characterized by a complex coupling between heat release, hydrodynamics and acoustics. The main focus is part of the talk is focused on accomplishing true predictivity: Dimension reduction approaches based on static manifolds – linear or non-linear – are not effective in predictive modeling of multi-scale problems with significant transport effects. To address this issue, we present an adaptive formulation in which the basis vectors and sampling points are adapted online using a novel non-local procedure, leading to ROMs that are demonstrated to be predictive in future state and parametric problems with negligible off-line training. We use this approach to develop a multi-fidelity framework in which component-level ROMs are trained on small domains, and integrated to enable full-system predictions in an affordable manner.
The second part of the talk will present advances in data-augmented computational physics, with the modeling of turbulent separated flows and wall heat transfer in hypersonic flows as a representative example. This approach has the following components: (1) Imposition of strictly necessary physical constraints; (2) Maintaining consistency between the learning and prediction environments; (3) Tightly-coupled inference and learning by constraining the augmentation to be learnable throughout the inference process; (4) Meticulous design of a parsimonious set of physics-informed, locally non-dimensional, and bounded features while promoting construction of a one-to-one features-to-augmentation map;(5) Localized learning to maintain explicit control over feature space to sensitize the augmentation function behavior only in the vicinity of available data.
Biosketch
Dr. Karthik Duraisamy is a Professor of Aerospace Engineering, Mechanical and Nuclear Engineering at the University of Michigan (U-M) where he also directs the Michigan Institute for Computational Discovery and Engineering (MICDE). His research interests are in various aspects of computational science and AI including data-driven and reduced order modeling, statistical inference, numerical methods and Generative AI for science. He is also the director of the multi-university Air Force Center of Excellence on Reduced Order modeling and PI of the U-M–Los Alamos Center on Advanced Computational Sciences. He is also the founder and chief scientist of the silicon-valley-based startup Geminus.AI, which is focused on developing digital twins for industrial processes.
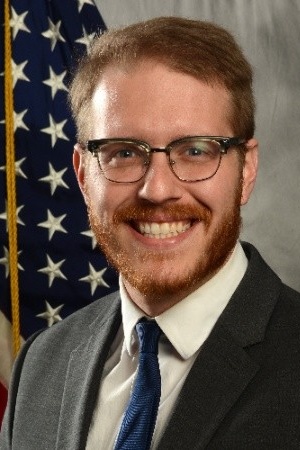
Brett Bornhoft
Research Aerospace Engineer, High-Speed Systems Division
Air Force Research Laboratory
Aircraft icing aerodynamics: relevant length scales for LES of real and artificial ice shapes
October 24, 2024
Predicting the aerodynamic performance of aircraft in icing conditions is crucial for ensuring flight safety. Modeling icing and its effect on aerodynamics has recently garnered attention in academia and industry due to the changes to the Code of Federal Regulations (CRF) in 2007. These changes require transport-category airplanes to demonstrate equivalent handling and performance in both icing and non-icing conditions. This has motivated airplane designers to include icing effects in the first stage of the design process.
Encouraged by recent studies using LES that demonstrate the ability to predict stall characteristics on full aircraft with smooth wings at an affordable cost (Goc et al. FLOW, 2021), this study seeks to apply this methodology to icing conditions. Using laser-scanned, detailed representations of the icing geometries, as well as artificially generated surfaces, wall-modeled LES calculations are conducted to compare integrated loads against experimental measurements in a variety of icing conditions, including glaze, rime, and scallop ice formations. This lecture will examine the necessary length scales for simulating iced airfoils, emphasizing the importance of resolving key ice shape features for accurate aerodynamic predictions. We will explore scenarios where resolving large-scale features is sufficient, as well as modeling approaches for situations where roughness length scales are sub-grid. The implications of removing some or all roughness scales, as is common with artificial ice shapes, will also be discussed. These modeling efforts provide a roadmap for the application of WMLES in the aircraft icing community and highlight its ability to predict the complex effects of ice shapes on aerodynamic performance.
Biosketch
Dr. Brett Bornhoft is a Research Aerospace Engineer at the Air Force Research Laboratory's High-Speed Systems Division where he has led efforts on code credibility and compressible multiphase flow modeling. He received his B.S. in Aerospace Engineering Technology from Middle Tennessee State University, his M.S. in Aerospace Engineering from North Carolina State University, and his Ph.D. in Mechanical Engineering from Stanford University working at the Center for Turbulence. He is a recipient of a Department of Defense SMART scholarship. His research focuses on computational fluid dynamics for various applications including compressible multiphase flows, high-speed air-breathing propulsion, aircraft icing, and rough-wall turbulent flows.
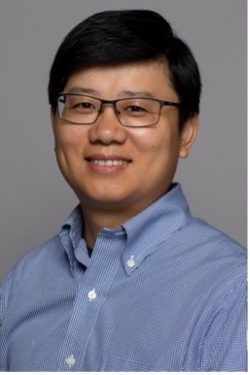
Binbin Wang
Associate Professor, Department of Civil and Environmental Engineering
University of Missouri
Dynamical behavior of hydrocarbon bubbles from natural marine seeps
October 31, 2024
Submarine hydrocarbon seeps are ubiquitous on the continental margins and are important to the global methane inventory. Quantitatively understanding transport of methane in the water column is important in assessing the supportive role of marine seeps in deep ocean microbial communities. It also helps predict methane emission from various depths in oceans. This presentation will discuss a combined effort of observation and modeling to understand dynamical behavior of hydrocarbon bubbles from deep-water natural seeps. Optical and acoustic imaging techniques were used to quantify characteristics of bubbles (size, shape, velocity) and their evolution with onset and dissociation of gas hydrate. This study reveals the detailed physical process of rising seep bubbles released within gas hydrate stability zone. This work is important to understand the role of natural seeps in the oceanic biochemical cycling.
Biosketch
Dr. Binbin Wang received his B.S. in Physics from Anhui Normal University, M.S. in Fire Science and Engineering from University of Science and Technology of China, and Ph.D. in Civil Engineering from University of Wisconsin–Milwaukee. His lab studies fundamental fluid flows and how they impact the natural and engineered aquatic environments. His research interests span a broad range of applications in environmental hydraulics, aquatic ecosystems, geophysics, and environmental health. Dr. Wang's research has been supported by National Science Foundation, U.S. Geological Survey, Office of Naval Research, Gulf of Mexico Research Initiative, and Missouri Department of Transportation. He was selected for 2022 early-career research fellowship of the National Academy of Sciences' Gulf Research Program.
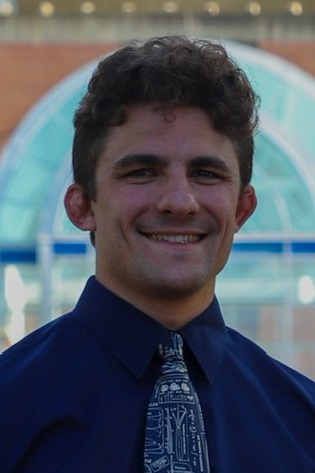
James Goldschmidt
Assistant Research Professor, Fluid Research Department
Penn State Applied Research Laboratory
Experimental investigation of the flow field and noise generated by urban air mobility rotors
November 7, 2024
Urban Air Mobility (UAM) presents a promising reality, where large-scale air taxi operations will be achieved using electric vertical take-off and landing vehicles (eVTOL). Unlike traditional rotorcraft the design strategy for these vehicles is to use multiple rotors with fixed-pitch rotor blades to reduce noise and increase vehicle stability. This design change alters the flow field surrounding the rotors and in turn the dominant noise sources, which need to be considered when determining community impact.
This seminar focuses on multiple experimental studies, which were conducted to understand the radiated noise and forces produced by both sub-scale UAM rotors in forward flight conditions. Noise directivities and scaling laws will be presented to highlight important noise sources for this new class rotors. Results from particle image velocimetry measurements of the rotor inflow and wake flow fields, will also be explored to show how experimental measurements can aid future noise modeling efforts. Specifically results from analytical tonal noise predictions will be presented, which combine low-fidelity force models with the measured rotor inflow to show that computationally efficient, accurate noise predictions, can be achieved with an accurate description of the rotor inflow.
Biosketch
Dr. James Goldschmidt is an Assistant Research Professor working in the Fluid Research Department at the Applied Research Lab at Penn State. He received his B.S. in Mechanical Engineering from The College of New Jersey in 2018, M.S. in Aerospace Engineering from The University of Florida in 2020, and Ph.D. in Aerospace Engineering from The University of Florida in 2023. His research focuses on experimental methods for fluid dynamics and aeroacoustics with focuses on low-order modeling of turbulent flow fields, flow control applications, rotorcraft aeromechanics, jet noise, and fluid structure interactions.
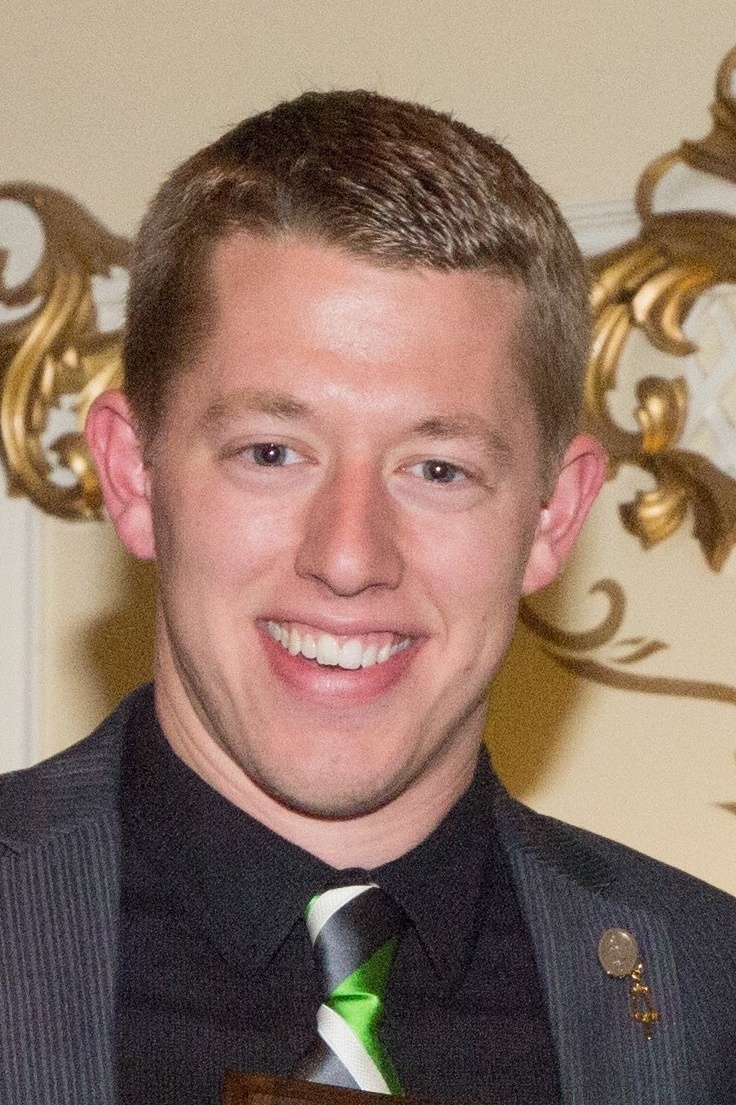
Christopher Douglas
Assistant Professor, Department of Mechanical Engineering and Materials Science
Duke University
Flash-back, blow-off, and symmetry breaking of premixed conical flames
November 14, 2024
Ongoing concerns about combustion-related greenhouse gas emissions have motivated substantial efforts to integrate alternative fuels such as hydrogen (H2) into existing energy infrastructure. Nonetheless, H2 is characterized by strong reactivity and remarkably high mass diffusion rates, leading to complex combustion dynamics that pose significant operational challenges for H2 as an alternative fuel. More specifically, lean premixed H2 flames feature extreme sensitivity to hydrodynamic stretch and intrinsic flame instabilities that drive intimate couplings between the flame and flow behavior. Nonetheless, the interaction and coupling of intrinsic flame dynamics with background flow gradients and boundary conditions remains poorly understood.
Motivated by these challenges, this seminar will present a global nonlinear bifurcation analysis of burner-stabilized laminar premixed conical flames in a fully-coupled framework. In it, the dynamics of flash-back, blow-off, and symmetry breaking are explored in mixtures with varying reaction rates and reactant diffusivities parameterized by the Damköhler number (Da) and the Lewis number (Le), respectively. Within this approach, the conical flame is identified as a steady solution of the low-Mach number, reacting Navier–Stokes equations that exists within a defined region of the (Le, Da) parameter plane. This region is bounded by curves of saddle–node (SN) bifurcations that correspond to spontaneous flame flash-back or blow-off, revealing how the parameters control these events. Further, the analysis shows that the conical flame experiences a sudden loss of axisymmetry via circle–pitchfork (CP) bifurcations in low-Le and high-Da parameter regimes. These bifurcations correspond to steady, three-dimensional global modes describing steady polyhedral or tilted flame structures, each associated with a distinct physical amplification mechanism and azimuthal periodicity. Finally, a center manifold reduction is used to elucidate the weakly-nonlinear dynamics governing the emergence of these structures and relate them to experimental observations. Overall, these results shed new light on the fundamental flame–flow interactions that control the behavior of burner-stabilized flames across a large range of Le and Da, including those relevant to H2 combustion.
Biosketch
Dr. Christopher Douglas is an incoming Assistant Professor in the Department of Mechanical Engineering and Materials Science at Duke University starting in January 2025. He earned his Ph.D. in Mechanical Engineering from Georgia Tech in 2021. Before starting at Duke, Christopher was a Marie Skłodowska-Curie Postdoctoral Fellow jointly at École Polytechnique and TU Munich and then a postdoctoral associate at the MIT Energy Initiative. His research primarily focuses on fluid and thermal sciences, with an emphasis on flow instability, combustion dynamics, and thermoacoustics. In his work, he develops theoretical and numerical methods to analyze, understand, and control the behavior of high-dimensional nonlinear systems encountered in engineering applications including turbines, rockets, and other energy systems, as well as in diverse natural phenomena such as atmospheric mixing and supernovae. His broader research interests include energy decarbonization and pollutant emissions abatement, with particular attention to potential alternative energy carriers like hydrogen and ammonia.
Christopher is actively seeking graduate students and postdocs for the 2025 calendar year. If interested, please reach out and detail your past and future interests.
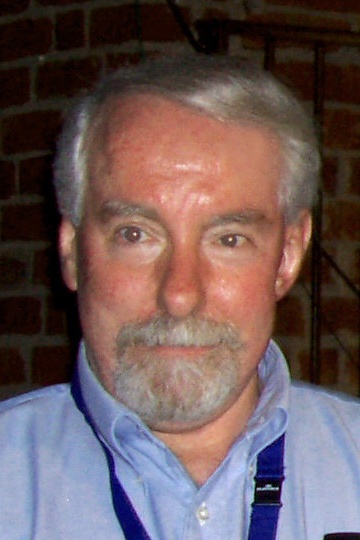
Gary Settles
Distinguished Professor Emeritus, Department of Mechanical Engineering
Pennsylvania State University
On BOS and its progenitor, the Background-Distortion Schlieren Technique
November 21, 2024
Background-Oriented Schlieren (BOS) is an experimental optical approach for imaging transparent phenomena in fluids (for example) by their distortion of a patterned background. It has been around for about 25 years and has attracted a lot of attention and many hundreds of publications. It's easy to do and lots of fun, it has some notable advantages over traditional optical instruments for flow visualization, and it also has a couple of drawbacks that need to be recognized. This talk is a broad overview of BOS, with some additional comments on refractive optical phenomena that can be seen by background distortion using only the unaided eye and one BOS variant that has become a popular table-top photography hobby.
Biosketch
Dr. Gary Settles earned his Ph.D. degree in Mechanical and Aerospace Engineering at Princeton University in 1976. After a research position at Princeton, he accepted an Assistant Professorship in Mechanical Engineering at Penn State University in 1983. In the ensuing 32 years at Penn State he educated 20 Ph.D. students and a great many Master's degree and undergraduate students, and he wrote or co-authored many publications on gas dynamics, optical flow diagnostics, shock wave/boundary-layer interactions, and industrial applications of compressible flow. Settles retired from Penn State in 2015. He is best known for his 2001 book on schlieren and shadowgraph techniques, for which a second edition is currently in preparation