FDRC Seminar Series
Each semester, FDRC invites speakers from across the United States and abroad to present their research on fluid dynamics to members of the Penn State community. Topics include fundamental research on turbulence, numerical methods for CFD, the development of experimental techniques, and engineering applications related to medicine, propulsion, combustion, and more.
Seminars are accompanied by complimentary coffee and donuts!
Fall 2022 Series
Seminars in this series are hosted every Thursday at 9:30 am in 125 Reber Building.
Schedule
Date | Speaker | Affiliation | Host |
---|---|---|---|
Sep. 1 | Sidharth GS | Iowa State University | Samuel Grauer |
Sep. 8 | Suo Yang | University of Minnesota, Twin Cities | Jacqueline O'Connor |
Sep. 15 | Alireza "Navid" Hooshanginejad | Brown University | Samuel Grauer |
Sep. 22 | Qi Wang | San Diego State University | Xiang Yang |
Sep. 29 | Ronald Joslin | National Science Foundation | Robert Kunz |
Oct. 6 | Theresa Saxton-Fox | University of Illinois at Urbana-Champaign | Xiang Yang |
Oct. 13 | Chi Young Moon | Argonne National Laboratory | Tamy Guimarães |
Oct. 20 | Arnold Mathijssen | University of Pennsylvania | Margaret Byron |
Oct. 27 | Tamer Zaki | Johns Hopkins University | Samuel Grauer |
Nov. 3 | James Braun | Purdue University | Tamy Guimarães |
Nov. 10 | Michael Roan | Pennsylvania State University | Zachary Berger |
Nov. 17 | APS Prep Session | Pennsylvania State University | |
Nov. 24 | Thanksgiving | ||
Dec. 1 | Michelle DiBenedetto | University of Washington | Margaret Byron |
Dec. 8 | Matthew Zahr | University of Notre Dame | David Williams |
Abstracts and Biosketches
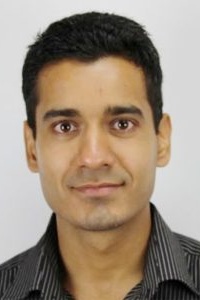
Sidharth GS
Assistant Professor, Department of Aerospace Engineering
Iowa State University
Modeling and simulations of variable-density flows
September 1, 2022
Density variations on the order of mean density are encountered in many technological and natural flows. These variations arise from compressibility, heat transfer, compositional inhomogeneity and/or reactions. Baroclinic torque from misaligned density and pressure gradients is an important vorticity production mechanism. (1) To explicitly represent baroclinic and dilatation effects on turbulent dissipation, we derive a new form of the governing equations for large eddy simulations, which meaningfully simplifies the closure problem as it involves only double correlations, and not triple and higher correlations that appear in the conventional equations. We demonstrate the use of the new equations in complex flows. (2) Non-local linear instabilities play a central role in transition to turbulence in hypersonic boundary layers. Using global tools, we analyze instabilities in separation zones and identify the role of centrifugal and baroclinic effects in the transition mechanism. (3) Diffusive models with an eddy viscosity are the norm in engineering turbulence closures. However, strong anisotropy and counter-gradient effects can require that simulations represent the turbulent processes more accurately. We discuss these aspects in the context of scalar transport by turbulence. All these topics account to put engineering simulations of high-speed aerodynamics and propulsion on a first principles basis.
Biosketch
Dr. Sidharth GS is an assistant professor in the Deptartment of Aerospace Engineering at Iowa State University. He was previously a postdoctoral researcher at the Los Alamos National Laboratory, where he worked on modeling mix and burn in multimaterial turbulence and separately on feature based dynamic reconstructions. He received his PhD at the University of Minnesota, specializing in computations of hypersonic flows and modeling variable-density subgrid effects relevant to supersonic combustion.
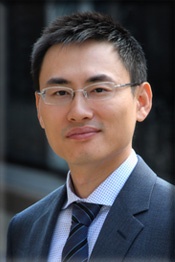
Suo Yang
Richard and Barbara Nelson Assistant Professor, Department of
Aerospace Engineering
University of Minnesota, Twin Cities
Multi-physics modeling for future propulsion and power systems: High-pressure phase separation and plasma assisted ignition
September 8, 2022
In the development of advanced propulsion and power systems, the growing concerns about emissions & efficiency and the pursuit of high speeds have pushed the systems to operate at extreme conditions. These conditions and the corresponding technical solutions often introduce more physics, which brings new challenges to modeling and simulation. In this presentation, I will talk about two examples of such multi-physics modeling from our recent works: (1) Advanced gas turbines and detonation engines operate at high pressures for high power density and efficiency. At such conditions, the injected multicomponent liquid propellants and fuel-air mixtures often go through trans-critical phase change. We developed a CFD framework based on the first-principle vapor-liquid equilibrium (VLE) theory to accurately predict high-pressure phase change, and demonstrated that phase separation can be triggered by either mixing or expansion waves. (2) Plasma assisted combustion (PAC) is a promising technology to enable ignition and stable combustion either at extreme conditions (e.g., high-speed flows in scramjets) or using low-reactivity fuels (e.g., carbon-free ammonia). We developed a series of 0D–3D CFD solvers to model PAC with multi-fidelity. Using our solvers, we figured out why plasma can surprisingly reduce the NOx emission of ammonia combustion and investigated how turbulence and pulsing frequency affect plasma assisted ignition.
Biosketch
Dr. Suo Yang is the Richard & Barbara Nelson Assistant Professor of Mechanical Engineering at the University of Minnesota, Twin Cities. During 2017–2018, Dr. Yang was a Postdoctoral Research Associate in Mechanical & Aerospace Engineering at Princeton University. He received Ph.D. (2017) and M.S. (2014) degrees in Aerospace Engineering, and another M.S. degree in Computational Science & Engineering (2015), all from Georgia Institute of Technology. He received a B.S. degree in Mathematics & Applied Mathematics from Zhejiang University in 2011. Dr. Yang's research focuses on the modeling and simulation of laminar and turbulent reacting flows, including combustion, plasma, particulate & multiphase flows, and hypersonics. He has been authored for more than 50 peer-reviewed journal articles and referred conference papers, in which he received Editor's Pick and Featured Article awards from Physics of Fluids and Combustion and Flame. Dr. Yang is a recipient of the DARPA Young Faculty Award (YFA) and the ONR Young Investigator Program (YIP) Award. His research is supported by NSF, ARL, ARO, DARPA, ONR, and ARPA-E. Dr. Yang is a Senior Member of AIAA and a committee member of three AIAA Technical Committees. He also serves as an active reviewer for many top-tier journals for which he received three Outstanding Reviewer Awards.
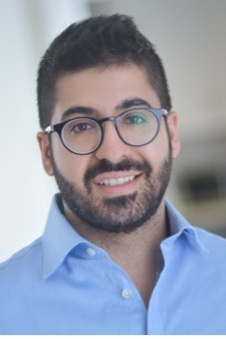
Alireza "Navid" Hooshanginejad
Hibbitt Postdoctoral Fellow, Center for Fluid Mechanics
Brown University
Sustainable cleaning of surfaces using air bubbles
September 15, 2022
Applying air bubbles to techniques for sanitizing surfaces has been growing rapidly in recent years from complex industrial machines to kitchen appliances. Specifically, air bubbles are proposed as a sustainable solution for cleaning biological surfaces to reduce high rates of foodborne illnesses globally. In the first half of this talk, I will discuss the fundamental source of such cleaning effects by discussing the shear stress exerted on the surface when a bubble bounces, and then slides along a tilted substrate. I will then show how we carry out cleaning experiments on controlled biological surfaces systematically. Our experimental results on bacteria-coated surfaces reveal that the optimum cleaning happens for a tilted surface of ~20°. To rationalize this finding, I will use numerical analysis and scaling arguments to explain how shear stress is maximized at a mid-value of tilting angles. In the second half of the talk, I will introduce an interesting phenomenon where bubbles of certain size show a backflipping behavior when they collide with a tilted surface. I will explain why bubbles exhibit this counter-intuitive behavior through experimental observations and reduced mathematical modeling. I will then discuss how this backflipping behavior of bubbles can be leveraged to enhance cleaning. Finally, time permitting, I will briefly show how applying sonic waves to bubbles also enhance cleaning by inducing infinite bouncing mode in them.
Biosketch
Alireza "Navid" Hooshanginejad is a Hibbitt Postdoctoral Fellow in the Center for Fluid Mechanics at Brown University. Navid received his B.Sc. (2014) in Mechanical Engineering from Sharif University of Technology in Iran, and his Ph.D. (2020) in Mechanical Engineering from the University of Minnesota. He has held a visiting Research Associate position at Flatiron Institute (2017), and a postdoctoral position in Biological and Environmental Engineering Department at Cornell University (2020–2022), before joining his current position at Brown University. Navid is an experimentalist and an applied mathematician working in the area of fluid mechanics and soft matter. His research interests include drops and bubbles, interfacial instabilities, pattern formation, and self-assembly with a focus on table-top experiments and mathematical modeling. Navid has been the recipient of the Graduate Teaching Fellowship from the University of Minnesota (2020), and the poster award from the Gordon Research Conference on Granular Matter (2022). Navid is one of the two nominated candidates in the current APS DFD election for the Early Career Member of the Executive Committee. He has organized multiple seminar series and panel discussions including the ME Connect Seminars at the University of Minnesota and the Cornell Fluids Seminars.
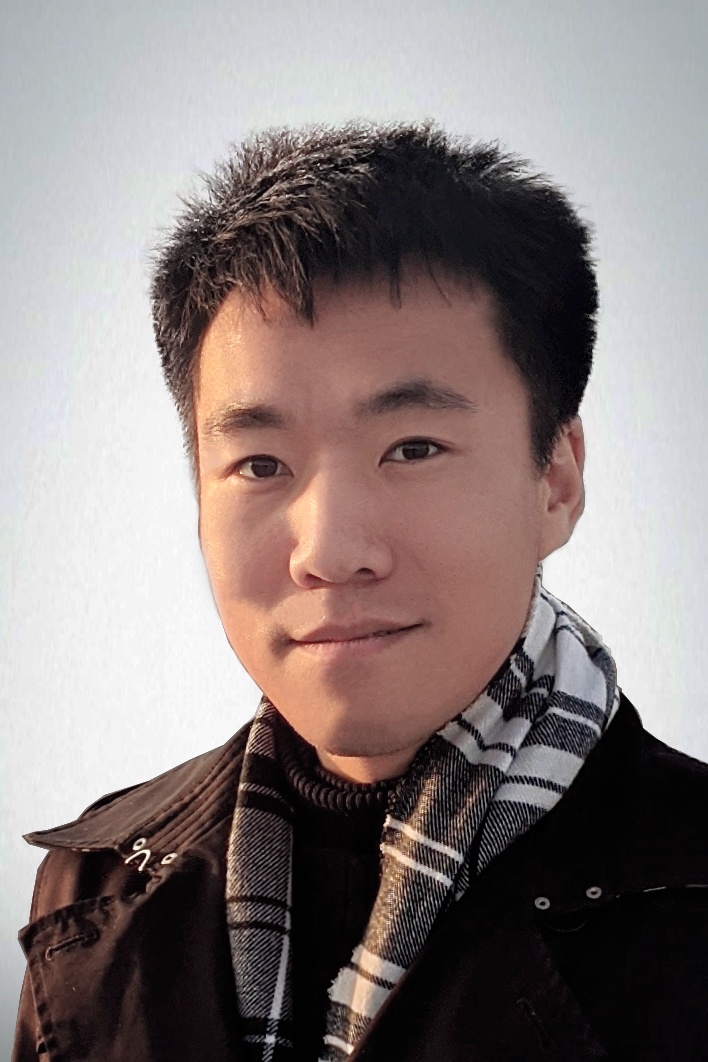
Qi Wang
Assistant Professor, Department of Aerospace Engineering
San Diego State University
On the interpretation of measurement in turbulent environments
September 22, 2022
Turbulence affects fluid flows across scales and application domains. The capability to accurately model and estimate information for turbulence is essential for understanding, predicting and controlling these chaotic flows. Two traditional approaches: laboratory experiments and numerical simulation can be combined to overcome their individual deficiencies. An approach that optimally infuses measurements from experiments into computational models is demonstrated, the realism and fidelity of computational models are increased and uncertainties mitigated. This data assimilation approach can be formulated as optimization problems, constrained by the nonlinear governing equations, and guided by gradients from their discrete adjoint. We demonstrate this adjoint-based optimization in two canonical scenarios: scalar source reconstruction and flow state estimation from limited measurements in a turbulent channel flow. Scalar source reconstruction focuses on finding the location of the source of passive pollutants transported in turbulent environments, which is an ill-posed problem with broad applications. The current study uses direct numerical simulations of both forward and adjoint scalar transport equations to identify the location and size of a source in turbulent channel flow based on sensor measurements downstream. We can reconstruct the spatial distribution of a steady source by doing forward and adjoint simulations repeatedly. The other application involves estimating the initial state of turbulent channel flow from surface measurements. The discrete adjoint operator is applied to perform a domain-of-dependence analysis of surface measurements. The analysis relies on the evaluation of the Hessian matrix of the cost function, in the vicinity of the true solution, and analysis of the Hessian eigen-spectra. The leading eigenmodes correspond to the highest sensitivity of measurements to the flow; these modes are concentrated in the near-wall region upstream of the sensor location. Their structure explains the fundamental difficulty of estimating the flow state from wall measurements.
Biosketch
Dr. Qi Wang's research exploits state-of-the-art mathematical and computational methods to predict and optimize complex turbulent flows, and to optimally place sensors and utilize measurements in numerical simulations. He made novel contributions to the interpretation of sparse measurements by exploiting the discrete adjoint operator in various scenarios, including passive scalar source localization from noisy sensor data and initial flow state reconstruction from wall measurements.
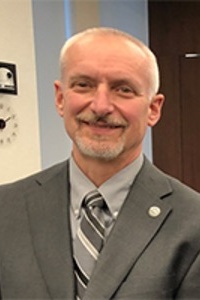
Ronald Joslin
Program Manager, Division of Chemical, Bioengineering, Environmental,
and Transport Systems
National Science Foundation
Engineering career: Industry, government, and university
…from student to professional… YOUR journey
September 29, 2022
You have completed the requirements for your B.S. in engineering. Now what? Or, you have conducted some research, passed some courses, and penned a thesis, and the university confers your M.S. and/or Ph.D. degree. Congratulations! But now what career path will you follow?
Your science or engineering career can evolve along different paths depending on your career goals. Is graduate school in your future? Should you pursue a post-doc position? Should you become a faculty member at a university, a research engineer at a company, or a research scientist at a government laboratory? The presentation will provide some perspectives on industry, government, and university career paths to consider as you begin your journey.
Biosketch
Dr. Ronald Joslin has been the Program Director of the National Science Foundation (NSF) Fluid Dynamics Program since 2016. He supports research in biofluids, micro-scale flows, transition & turbulence, non-Newtonian flows, geophysical fluids, and renewable energy. Teaming with AFOSR, he supports research in hypersonics, and, working with NASA/CASIS, he supports research on the International Space Station. He is an engineering representative on cross-foundational teams to support research in Artificial Intelligence (AI), machining learning (ML), advanced algorithms, and advanced measurement techniques.
Prior to NSF, Dr. Joslin was a Program Officer at the Office of Naval Research for 15 years where he managed Turbulence, Stratified Wakes, Submarine Maneuvering, Ocean Energy, Multi-Platform Interactions and Supercaviation Programs. Prior to ONR, Dr. Joslin was on the faculty at Penn State for 2 years, and he led active and laminar flow control teams and conducted research at NASA Langley for 10 years.
He is an Associate Fellow of the AIAA and a Fellow of the ASME. Throughout his career, he has mentored students, faculty, and peers and has volunteered to visit us today to talk about careers.
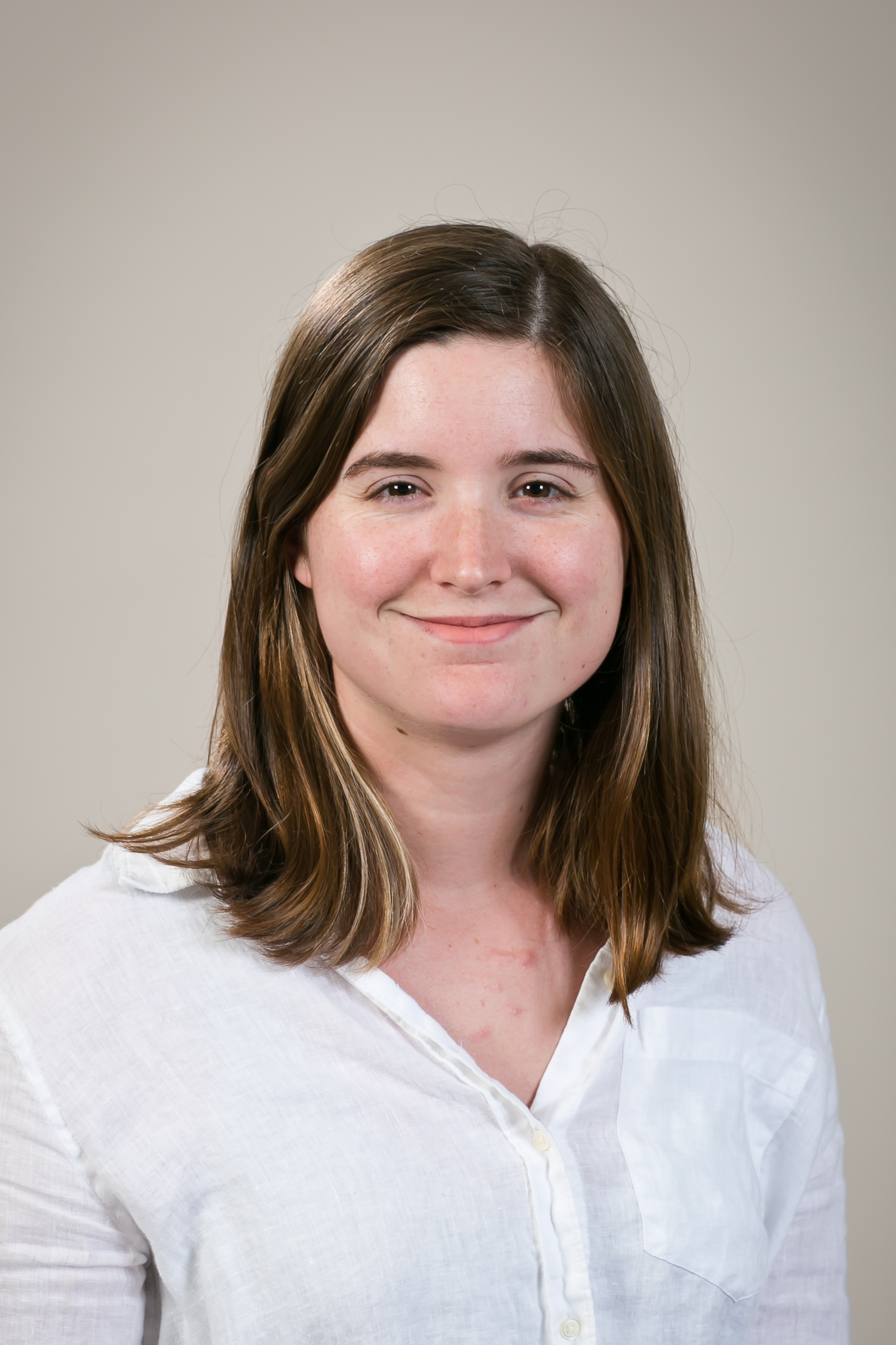
Theresa Saxton-Fox
Assistant Professor, Department of Aerospace Engineering
University of Illinois at Urbana-Champaign
History effects in turbulent boundary layers: Spatially and temporally varying pressure gradients
October 6, 2022
Wall-bounded turbulent flows are ubiquitous in transportation and energy technologies on curving bodies and in unsteady flows. Turbulence is complex even in "canonical" geometries (flat walls, straight pipes, and straight channels); geometry curvature and flow acceleration further complicate its study. In this talk, we discuss novel experimental facilities and results towards the systematic characterization of complex pressure gradient and curvature effects, including variable spatial and temporal history. The experiments are carried out using a variable-positioned ceiling and are measured using time-resolved, planar particle image velocimetry. Statistical results are shown for both statically held and dynamic pressure gradients. History effects (prior pressure gradients imposed upstream of the position of interest) and dynamic effects (a dependence on the rate of pressure gradient imposition) are both observed. A hypothesis to explain the observed history effects from a coherent structures perspective is suggested.
Biosketch
Theresa Saxton-Fox is an Assistant Professor of Aerospace Engineering at the University of Illinois at Urbana-Champaign. She received her master's and PhD from Caltech and did her postdoctoral research at Princeton University, prior to starting at the University of Illinois in January 2019. Her work focuses on wall-bounded turbulent flows with particular interests in nonlinear interactions, global unsteadiness, and curvature effects. She was awarded the Centennial prize for best thesis in the Mechanical and Civil Engineering department at Caltech in 2018 and the Young Investigator Program award from the Office of Naval Research in 2021.
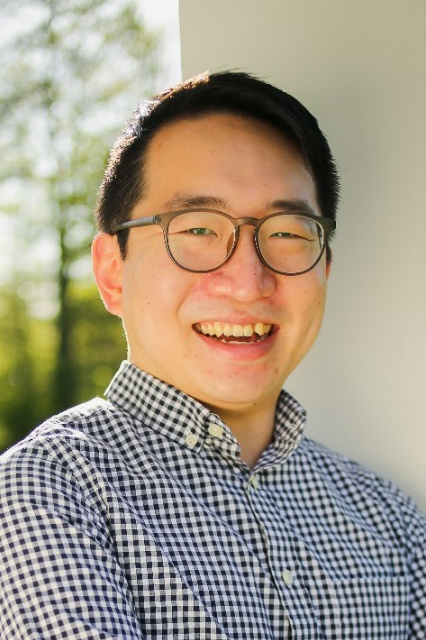
Chi Young Moon
Postdoctoral Appointee, Transportation and Power Systems Division
Argonne National Laboratory
Synchrotron-based X-ray diagnostics for fluid dynamics
October 13, 2022
Complex, multi-phase flows are critical to system performance in various applications, including in automotive and aerospace. For situations where optical diagnostics are difficult to apply, such as internal flows or in near-surface regions, X-rays are useful due to their ability to optically penetrate opaque materials. Synchrotron light sources, such as Argonne National Laboratory's Advanced Photon Source, produce X-rays with orders of magnitude higher flux than laboratory sources, enabling unique diagnostic capabilities for multi-phase fluid dynamics. In this presentation, we will provide a brief overview of X-ray diagnostic techniques for fluid mechanics research at Argonne, as well as few recent examples from our experimental campaigns highlighting our capabilities.
Biosketch
Dr. Chi Young Moon is a postdoctoral appointee at Argonne National Laboratory's Transportation and Power Systems Division. He received his B.S. in Aerospace Engineering from Illinois Institute of Technology and his Ph.D. in Aerospace Engineering from Virginia Tech. As a member of Argonne's X-ray Fuel Spray team, he conducts research utilizing X-ray diagnostics for automotive and aerospace applications of multi-phase flows at the Advanced Photon Source.
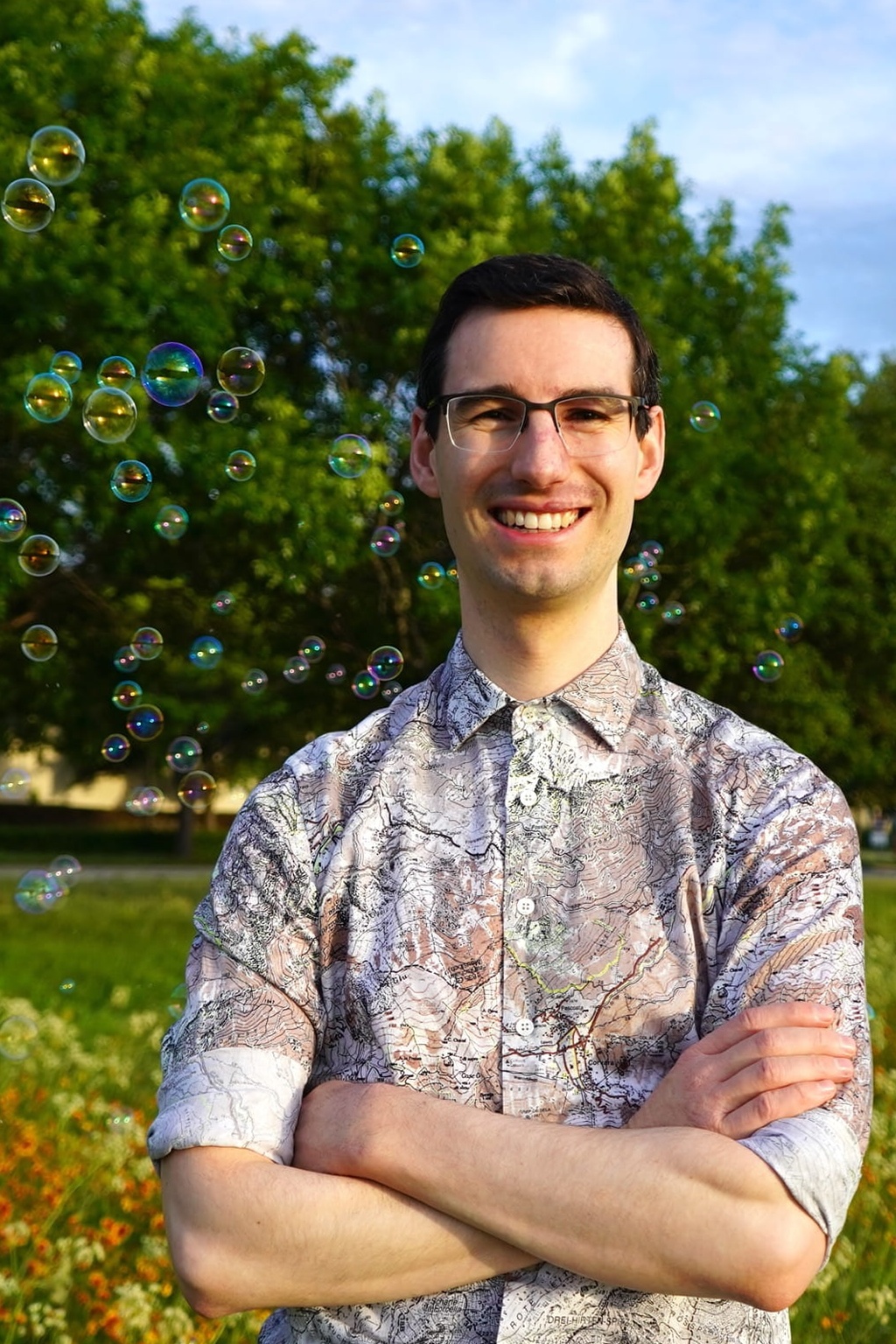
Arnold Mathijssen
Assistant Professor, Department of Physics and Astronomy
University of Pennsylvania
Transport and delivery by living materials
October 20, 2022
One of the major challenges in robotics is controlling transport and micromanipulation by active and adaptive materials. Existing delivery technologies often suffer from limited navigation control, low speeds, and proneness to environmental disturbances. Biology often solves these problems by collectively organizing actuation at the microscale. For example, pathogens are removed from our lungs by an active carpet of cilia. Inside these cilia, in turn, microtubules form highways for molecular motors. In this talk, I will present developments in the microfabrication of "artificial cilia" and "artificial microtubules". We designed amphibious cilia that can transport both liquids and dry objects. These carpets can sort particles by size and by shape using a crowd-surfing effect. We also designed magnetic microtubules, structured microfibers that rapidly guide particles through flow networks such as the cardiovascular system. These works offer unique strategies for robust microscale delivery, but equally shed light on non-equilibrium effects in biological transport processes.
Biosketch
Dr. Arnold Mathijssen was named '30 under 30' by Scientific American and was awarded the Sir Sam Edwards PhD Thesis Prize for his work in group of Julia Yeomans FRS at the University Oxford (2016). Supported by an HFSP cross-disciplinary fellowship, he moved to the lab of Manu Prakash at Stanford University, where the American Physical Society presented him the Charles Kittel Award (2019). He is now Assistant Professor of Physics & Astronomy at UPenn, and guest editor of the journal Physics of Fluids.
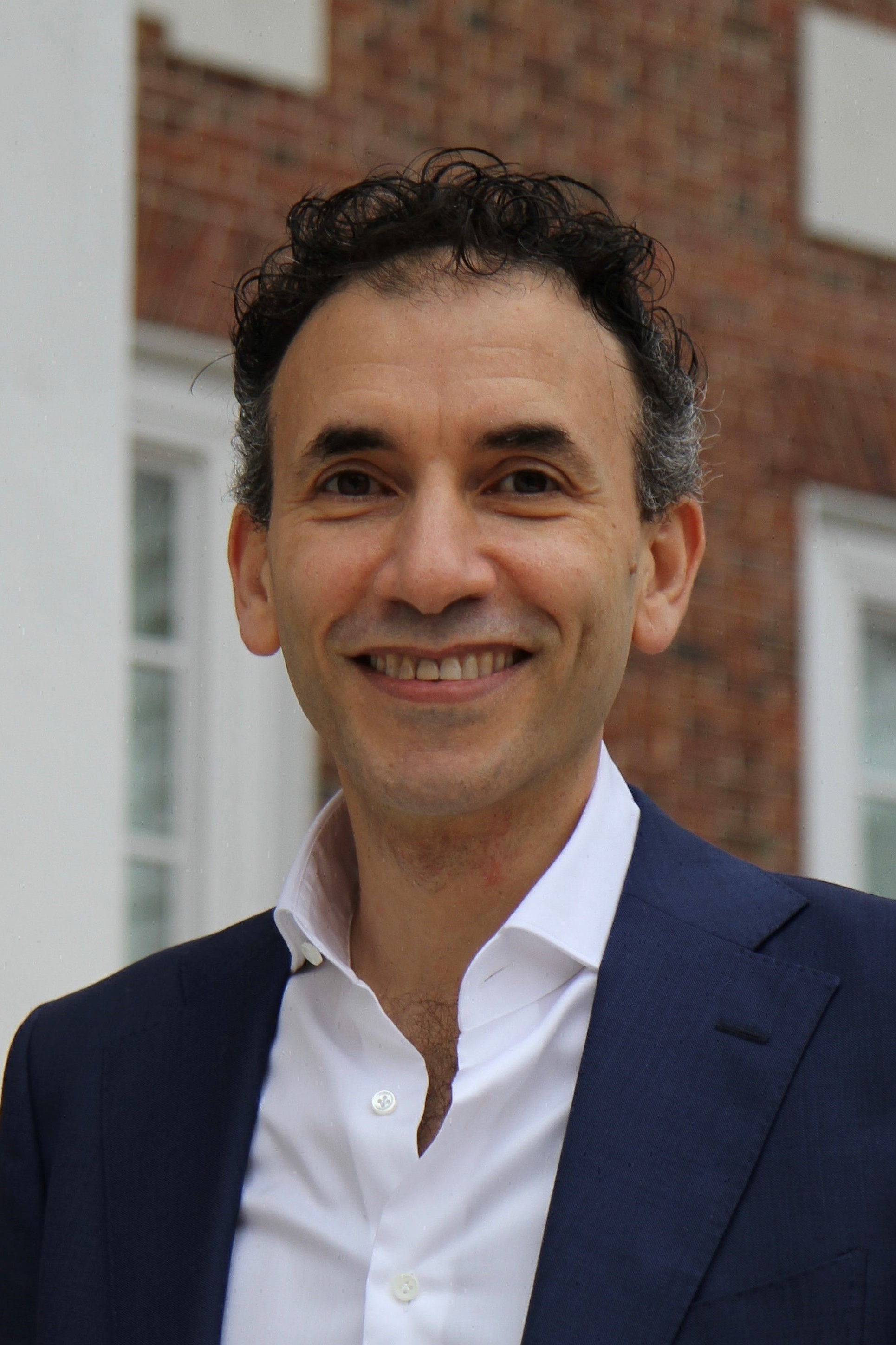
Tamer Zaki
Professor, Department of Mechanical Engineering
Johns Hopkins University
Synchronization, estimation and observability of wall turbulence
October 27, 2022
High-fidelity simulations of turbulence provide non-intrusive access to all the resolved flow scales and any quantity of interest. However, simulations often invoke idealizations that compromise realism (e.g. truncated domains and modelled boundary conditions). Experiments, on the other hand, examine the true flow with less idealizations, but they continually contend with limited spatio-temporal sensor resolution and the challenge of directly measuring quantities of interest. By assimilating observations, however scarce, in simulations, we can leverage the advantages of both approaches and mitigate their respective deficiencies. The simulations thus achieve higher level of realism by tracking the true flow state, and we can probe any flow quantity of interest at higher resolution than the original measurements. The data-assimilation problem is formulated as a nonlinear optimization, where we seek the flow field that satisfies the Navier–Stokes equations and optimally reproduces available data. In this framework, measurements are no longer mere records of instantaneous, local flow quantities, but rather an encoding of the antecedent flow events that we decode using the governing equations. Chaos plays a central role in obfuscating the interpretation of the data. Measurements that are infinitesimally close may be due to entirely different earlier conditions—a dual to the famous butterfly effect. We will examine several data-assimilation problems in wall turbulence and establish the minimum resolution of measurements for which we can accurately reconstruct all the missing flow scales. We will highlight the roles of the Taylor microscale and the Lyapunov timescale and discuss the fundamental difficulties of predicting turbulence from limited observations.
Biosketch
Dr. Tamer Zaki is a professor in the Department of Mechanical Engineering at Johns Hopkins University (JHU). He received his Ph.D. (2005) in Flow Physics and Computational Engineering from Stanford University. In 2006 he joined Imperial College London where he was an Assistant and subsequently Associate Professor of Mechanical Engineering. He has been at Johns Hopkins since 2013. Zaki's research applies high-fidelity simulations for the prediction of transitional and turbulent shear flows, in both Newtonian and complex fluids. He has developed state-of-the-art data assimilation techniques for the interpretation of scarce flow measurements, and effective optimization strategies for sensor placement in uncertain environments. Zaki has garnered several recognitions including the Office of Naval Research Young Investigator Award and the William H. Huggins Excellence in Teaching Award. He is a member of the JHU Center for Environmental & Applied Fluid Mechanics, the Institute for Data Intensive Engineering & Science, and the American Physical Society. He serves on the Editorial Advisory Board of Flow, Turbulence and Combustion and is an Associate Editor of the Journal of Fluid Mechanics.
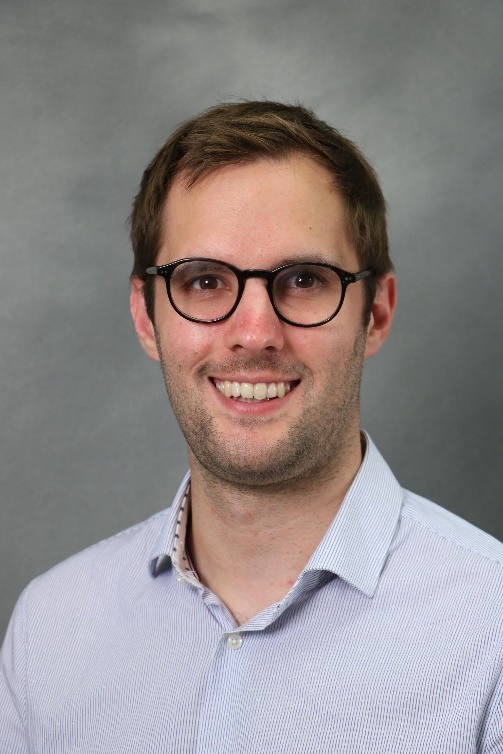
James Braun
Research Assistant Professor, School of Mechanical Engineering
Purdue University
The bladeless turbine, a clean expansion device for rotating detonation combustors
November 3, 2022
In the past decade, pressure gain combustion research has promised over ten percentage-points of increase in power plant thermal efficiency. The impact of a mere two percent increase in fuel efficiency for airplane engines would save over one billion dollars for the United States per year, not to mention potential reductions in size, weight, complexity, and cost of engines. However, to realize such potential gains, the turbine must be effectively coupled with the rotating detonation combustor (RDC), whose exhaust conditions differ substantially from current state of the art turbines. The RDC utilizes isochoric combustion through detonation waves traveling at supersonic speeds, and is suitable for many types of fuels, especially carbon-free fuels such as hydrogen. We will first go over the characterization of rotating detonation combustors (RDCs). Afterwards a new type of turbine, the wavy-shaped bladeless turbine is introduced. This is an energy conversion unit for the combined power extraction and thrust increase which can be employed in high subsonic to hypersonic flows, and especially suitable for RDCs. The seminar will include the advantages and current state of bladeless turbines, the design procedure, the performance characteristics, and an experimental validation in a supersonic wind tunnel through high-frequency probe-based and optical measurements.
Biosketch
Dr. James Braun graduated from the KU Leuven (Belgium) as mechanical engineer. He performed a research master's degree at the von Karman institute for fluid dynamics (Belgium) in 2015 within the Aerospace and Aeronautics department. His thesis was on the 'Characterization of complex multi-physics flow in rotating detonation engines' for which he won the Prince Alexandre of Belgium Award for the Best Presentation. He obtained his PhD from Purdue University (USA) in 2019 entitled 'power harvesting from shock waves: the axial bladeless turbine'. In 2018, he was awarded with the AIAA Gordon C Oates Air Breathing propulsion graduate award. He was an ORISE postdoctoral researcher in 2019 and is currently a research assistant professor at Purdue University within the PETAL group (Purdue Experimental Turbine Aerothermal Lab).
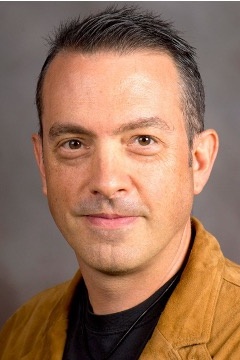
Michael Roan
Professor, Department of Mechanical Engineering
Pennsylvania State University
Industrial and national security applications of acoustics, signal processing, and immersive reality research
November 10, 2022
The Acoustics, Signal Processing, and Immersive Reality (ASPIRe) Lab at Penn State strives to make advancements across a wide array of application areas, but with core strength in the underlying fundamentals in Acoustics/Vibrations and Statistical Signal Processing. This presentation will give a broad overview of the research efforts of the ASPIRe Lab past, present, and future, with emphasis on four major areas: Microphone Array Technology, Acoustics/Vibration-Based Machinery Health Monitoring, 3D Immersive Audio, and Electric Vehicle Safety. Special emphasis will be given to past and current projects that highlight potential collaboration across multiple research units at Penn State.
Biosketch
Dr. Michael Roan completed his MS degree in the Graduate Program in Acoustics at Penn State, after which he went to work at Noise Cancellation Technologies, Inc. in Linthicum, Maryland, and then moved on to Westinghouse ESG/Alliant Tech Systems. Having spent several years in industry, Dr. Roan decided to return to graduate school to finish his doctorate in Acoustics. While working full-time at the Applied Research Laboratory at Penn State, he received his PhD in Acoustics from Penn State in 1999 under the supervision of Dr. Leon Sibul. During the 2004 academic year, Dr. Roan was invited to work at Princeton University as a visiting fellow in the Electrical Engineering Department under the mentorship of Professor Stuart C. Schwartz. Dr. Roan's time at Princeton motivated him to pursue a career in academia, and in 2005 he moved to Virginia Tech for 16 years. In 2022, Dr. Roan returned to Penn State joining the ME department and the Applied Research Laboratory and has been working in the areas of Acoustics and Statistical Signal Processing, primarily in defense- and security-related areas. His recent interests include full 3D audio immersion for electric vehicle safety applications, virtual machinery prototyping, and theatre/concert sound.
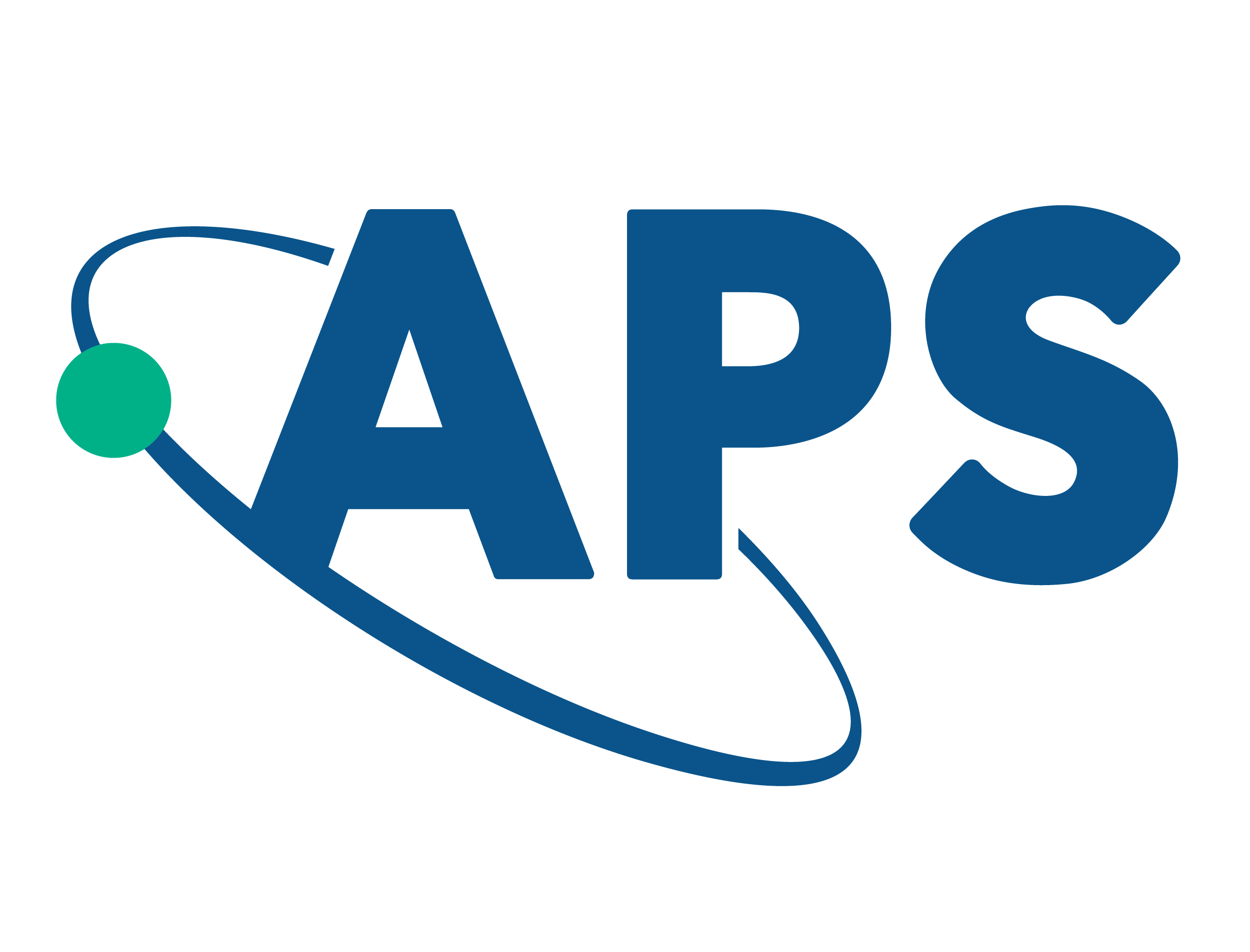
APS Prep Session
November 17, 2022
Modal analysis of a shear layer in high-supersonic cavity flows using data-driven and operator-based resolvent analysis
Aravinth Sadagopan, Department of Aerospace Engineering
Shear layer oscillations (SLO) are common phenomena in aerospace applications inducing unfavorable acoustic radiation and structural fatigue. SLO driving mechanism over open cavity flows at subsonic and supersonic speeds have been well studied. However, for SLO over a cavity in high supersonic flows, the stability properties are relatively unexplored, i.e., for flow regimes beyond Mach 3. The classical Rossiter model fails to predict the SLO characteristics. Therefore, in this work resolvent analysis has been employed to identify the dominant dynamic mechanisms and modal characteristics of self-sustained SLO at high Mach numbers. In particular, the resolvent analyses are performed with both the data-driven and operator-based frameworks. The comparisons between both implementations will be highlighted for the resolvent modes and spectra. We will also discuss the influence of the baseflows due to the differences in the solver fidelity. With a series of implicit large-eddy simulations at Mach numbers 2 to 5, we observe a weak coupling between standing waves within the cavity and SLO. The strong compression/expansion waves on the shear layer and cavity wall reflected Mach waves significantly contribute to the SLO characteristics.
The role of droplet modeling in a five-field model of ice accretion
Arshia Merdasi, Department of Mechanical Engineering
The impingement and ice accretion of droplets on wing surfaces presents a serious challenge to aircraft safety and efficiency. We have developed an Eulerian multiphase model of icing. Five fields are transported including compressible air, water-vapor, droplets, film and ice. An immersed boundary method is employed to accommodate ice accretion. In this talk we will focus on droplet dynamics and heat and mass transfer modeling. Specifically, we have incorporated models for film deposition due to impaction and turbulent diffusion mechanisms, splashing, bouncing and re-entrainment, droplet heat transfer and attendant mass transfer evaporation, condensation, and freezing. We present predictions of collection efficiency on different airfoils to validate the numerous interfacial mass and dynamics models involved in the deposition process. It is found that for airfoil ice-shape modeling at relevant atmospheric conditions, it is critical to incorporate accurate modeling of the impaction, diffusion and splash/bounce elements of deposition processes, whereas re-entrainment is not as dynamically important. Also, the roles of droplet initial conditions (liquid, partially frozen, ice) and size distributions are explored in the context of their impact on ice shape.
Simulating the flow of human crowds
Eric Anderson, Department of Mechanical Engineering
The simulation of high-density crowds is used to design a safer building layout to reduce the risks of suffocation in crowds. Due to the intelligence of people, crowds are typically thinking fluids, decreasing the possibility to simulate accurately what individuals may do within the crowd. If the density of the crowd is very high and the goal of the majority is similar, then it is feasible to treat a high-density crowd as a Newtonian fluid. This simulation can then locate the high-pressure zones and the layout can be adjusted to reduce the pressure difference to reduce the risk of death by suffocation or the displeasure of individuals during travel. The effects of Stokes flow are also seen in some of the simulations.
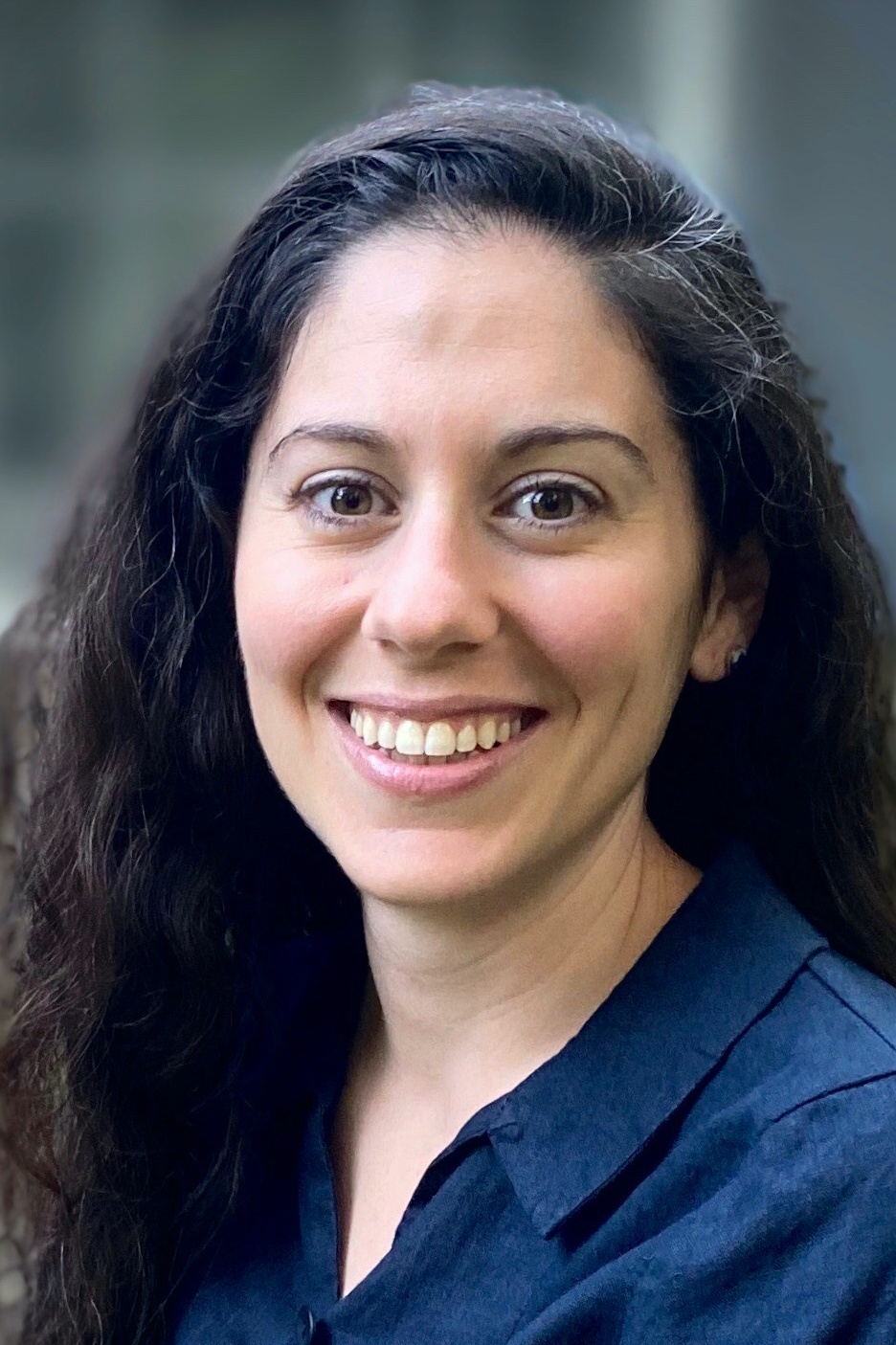
Michelle DiBenedetto
Assistant Professor, Department of Mechanical Engineering
University of Washington
Microplastics at the ocean surface: waves, turbulence, and particles
December 1, 2022
Plastic pollution poses a critical threat to the world's oceans, but critical gaps in knowledge surrounding plastic fate and transport impede remediation and prevention efforts. Much of the plastic in the ocean exists as microplastics. Predicting the behavior of microplastics is non-trivial for two primary reasons: their physical properties (size and density) are fundamentally different from traditionally studied environmental particles like sediment and bubbles, and complex interactions among waves, turbulence, and particle inertia in the ocean surface boundary layer (where most microplastics reside) are not well-understood, especially for buoyant particles such as microplastics. In this talk, I will discuss the importance of surface waves in predicting the transport and distribution of microplastics. I will present results from both an analytical study and laboratory experiments of particles in wavy flows, and discuss these in the context of microplastic transport in the ocean.
Biosketch
Dr. Michelle DiBenedetto is an Assistant Professor at University of Washington Seattle in the Mechanical Engineering department. She received her B.S. from Cornell University in 2014 in Environmental Engineering, and her Ph.D. from Stanford University in 2019 in Civil & Environmental Engineering where she studied the behavior and transport of non-spherical particles in surface gravity waves. For her dissertation work, she was awarded the Andreas Acrivos Dissertation Award in Fluid Dynamics from the American Physical Society. Prior to her current appointment, she was a postdoctoral scholar at Woods Hole Oceanographic Institution in the biology and physical oceanography departments. Her lab at University of Washington uses laboratory experiments, observations, and mathematical modelling to study problems at the intersection of environmental fluid mechanics and particle-laden flows.
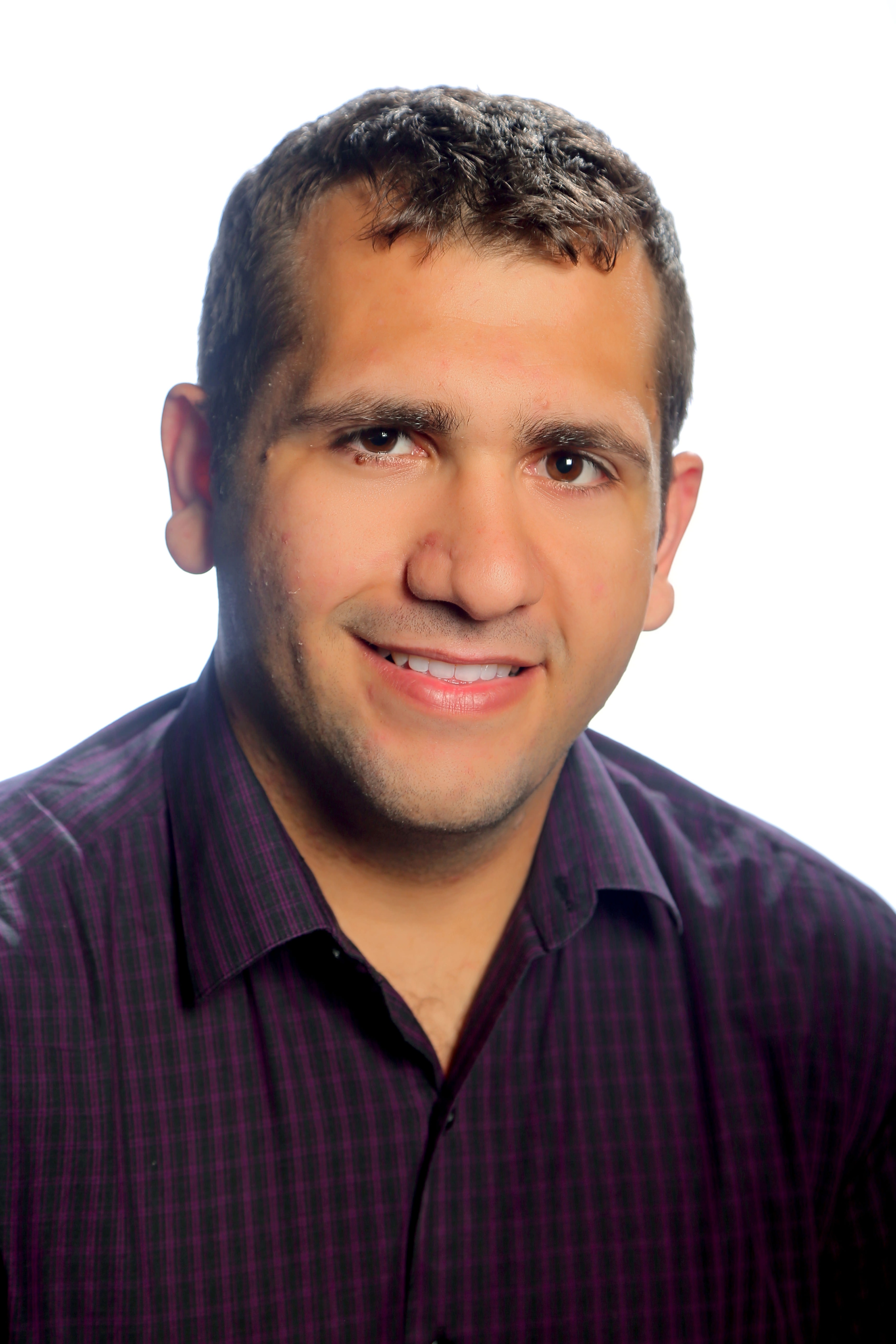
Matthew Zahr
Assistant Professor, Department of Aerospace and Mechanical
Engineering
University of Notre Dame
Simulation of shock-dominated flows using high-order implicit shock tracking
December 8, 2022
High-order implicit shock tracking (fitting) is a new class of numerical methods to approximate solutions of conservation laws with non-smooth features, e.g., contact lines, shock waves, and rarefactions. These methods align elements of the computational mesh with non-smooth features to represent them perfectly, allowing high-order basis functions to approximate smooth regions of the solution without the need for nonlinear stabilization, which leads to accurate approximations on traditionally coarse meshes. The hallmark of these methods is the underlying optimization formulation whose solution is a feature-aligned mesh and the corresponding high-order approximation to the flow, which circumvents the geometric complexity of traditional shock fitting approaches. In this talk, I will introduce the High-Order Implicit Shock Tracking method and its application to several shock-dominated flows.
Biosketch
Dr. Matthew Zahr is an assistant professor in the Department of Aerospace and Mechanical Engineering at the University of Notre Dame. He received his PhD in Computational and Mathematical Engineering from Stanford University in 2016 and from 2016–2018 was the Luis W. Alvarez Postdoctoral Fellow in the Department of Mathematics at Lawrence Berkeley National Laboratory.