FDRC Seminar Series
Each semester, FDRC invites speakers from across the United States and abroad to present their research on fluid dynamics to members of the Penn State community. Topics include fundamental research on turbulence, numerical methods for CFD, the development of experimental techniques, and engineering applications related to medicine, propulsion, combustion, and more.
Seminars are accompanied by complimentary coffee and donuts!
Spring 2023 Series
Seminars in this series are hosted every Thursday at 9:30 am in 262 Willard Building.
Schedule
Date | Speaker | Affiliation | Host |
---|---|---|---|
Jan. 12 | Clarence Rowley | Princeton University | Jacqueline O'Connor |
Jan. 19 | Joseph Kuehl | University of Delaware | James Coder |
Jan. 26 | Nicholas Ouellette | Stanford University | Margaret Byron |
Feb. 2 | Xiaofeng Liu | Pennsylvania State University | Robert Kunz |
Feb. 9 | SciTech Blitz Talks | Pennsylvania State University | |
Feb 16. | Matthew Berry | Raytheon Missiles & Defense | Zachary Berger |
Feb. 23 | Todd Lowe | Virginia Tech | Tamy Guimarães |
Mar. 2 | Jeffrey Sutton | Ohio State University | Samuel Grauer |
Mar. 9 | Spring Break | ||
Mar. 16 | Christopher Crowley | Johns Hopkins University | Samuel Grauer |
Mar. 23 | Casey Harwood | University of Iowa | Tamy Guimarães |
Mar. 30 | David Darmofal | Massachusetts Institute of Technology | David Williams |
Mar. 31 | Azar Panah | Penn State Berks | Robert Kunz |
Apr. 6 | James Brasseur | University of Colorado Boulder | Robert Kunz |
Apr. 13 | Jane Bae | California Institute of Technology | Xiang Yang |
Apr. 20 | Jifu Tan | Northern Illinois University | Melissa Brindise |
Apr. 27 | Andrew Banko | United States Military Academy, West Point | Samuel Grauer |
Abstracts and Biosketches
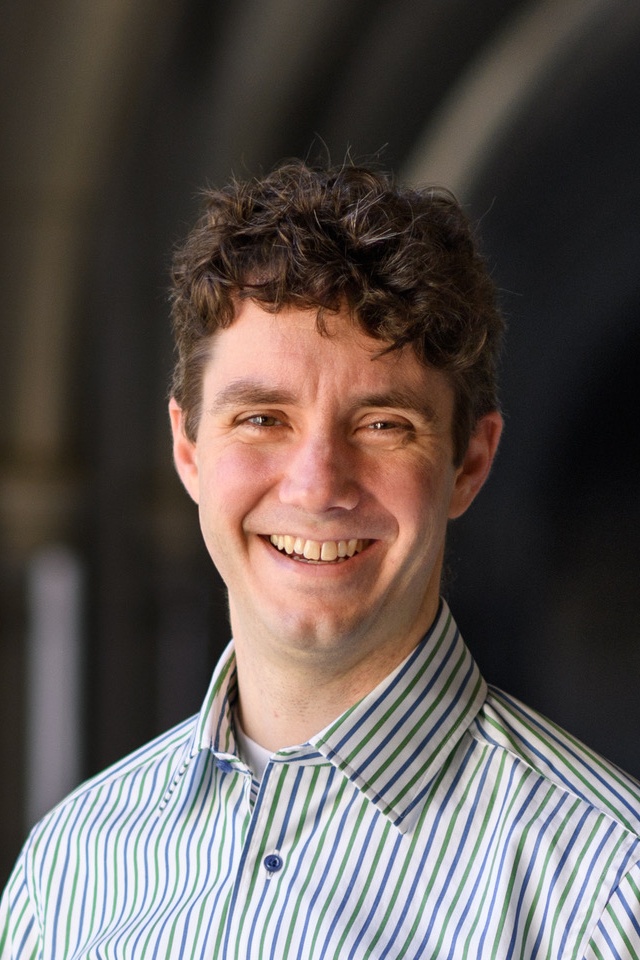
Clarence Rowley
Professor, Department of Mechanical and Aerospace Engineering
Princeton University
Data-driven modeling of fluids
January 12, 2023
Fluid flows can be extraordinarily complex, and even turbulent, yet often there is structure lying within the apparent complexity. Understanding this structure can help explain observed physical phenomena, and can help with the design of control strategies in situations where one would like to change the natural state of a flow. This talk addresses techniques for obtaining simple, approximate models for fluid flows, using data from simulations or experiments. We discuss a number of methods, including principal component analysis, balanced truncation, and Koopman operator methods, and focus on a new method for optimizing projections of nonlinear systems. We apply these techniques to several flows with complex behavior, including a transitional channel flow and an axisymmetric jet.
Biosketch
Dr. Clancy Rowley is the Sin-I Cheng Professor of Engineering Science in the Department of Mechanical and Aerospace Engineering at Princeton and is an associated faculty in the Program in Applied and Computational Mathematics. He received his undergraduate degree from Princeton and his doctoral degree from Caltech, both in Mechanical Engineering. He has received several awards, including an NSF CAREER Award and an AFOSR Young Investigator Award, and he is a Fellow of the American Physical Society. His research interests lie at the intersection of dynamical systems, control theory, and fluid mechanics, and focus on reduced-order models suitable for analysis and control design.
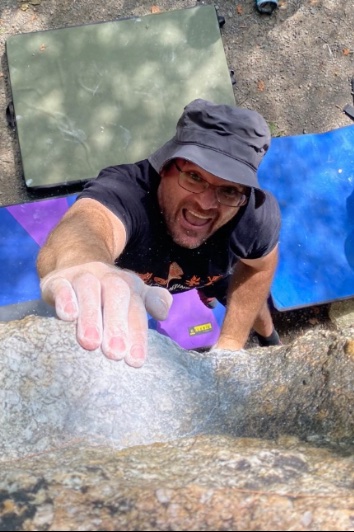
Joseph Kuehl
Associate Professor, Department of Mechanical Engineering
University of Delaware
Progress towards understanding the physics of high-speed boundary layer instability
January 19, 2023
Over the last decade, significant advances in our understanding of hypersonic boundary layer stability have been made. Many of these advances have come via developments of new experimental capabilities, advances in both computing power and code development, and through flight testing. In this talk, improvements to our understanding based in theoretical advances will be emphasized as well as their relationship with the experimental and computational advances. The discussion will focus on: The recognition that vortex modes evolve along paths defined by the crossflow inflectional profile. The development of a wave packet formulation for the Nonlinear Parabolized Stability Equations method. A thermoacoustic interpretation of second mode instability. A clarification of the fundamental dynamics of first mode instability. Consequences of these advances will be discussed in relationship to multi-mode/mechanism instability physics and nose bluntness effects.
Biosketch
Dr. Joseph Kuehl is an Associate Professor at the University of Delaware in the Mechanical Engineering Department. He holds Ph.D.s in Physical Oceanography and Mechanical Engineering from the Graduate School of Oceanography and Department of Mechanical, Industrial, and Systems Engineering at the University of Rhode Island (2009). His research interests include geophysical fluid dynamics (gap-leaping boundary currents, geophysical boundary layer dynamics and transport phenomena), hypersonic boundary-layer stability (numerical laminar-turbulent transition) and nonlinear vibrations (time series analysis, modal decomposition techniques and finite time invariant manifold analysis). He was the recipient of the AFOSR Young Investigator Award (2015) for his hypersonic boundary layer stability and transition research, participates in the NATO STO AVT hypersonic vehicle working groups (240, 190, 346) and was a member of the National Academy of Science Committee on Advancing Understanding of the Gulf of Mexico Loop Current Dynamics.
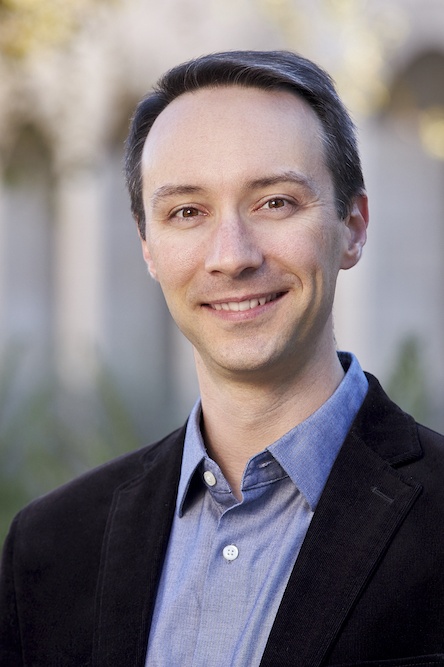
Nicholas Ouellette
Professor, Department of Civil and Environmental Engineering
Stanford University
Tensor geometry in the turbulent cascade
January 26, 2023
Perhaps the defining characteristic of turbulent flows is the directed flux of energy from the scales at which it is injected into the flow to the scales at which it is dissipated. Often, we think about this transfer of energy in a Fourier sense; but in doing so, we obscure its mechanistic origins and lose any connection to the spatial structure of the flow field. Alternatively, quite a bit of work has been done to try to tie the cascade process to flow structures; but such approaches lead to results that seem to be at odds with observations. I will discuss what we can learn from a different way of thinking about the cascade, this time as a purely mechanical process where some scales do work on others and thereby transfer energy. This interpretation highlights the fundamental importance of the geometric alignment between the turbulent stress tensor and the scale-local rate of strain tensor, since if they are misaligned with each other, no work can be done and no energy will be transferred. We find that (perhaps surprisingly) these two tensors are in general poorly aligned, making the cascade a highly inefficient process. Our analysis indicates that although some aspects of this tensor alignment are dynamical, the quadratic nature of Navier–Stokes nonlinearity and the embedding dimension provide significant constraints, with potential implications for turbulence modeling.
Biosketch
Dr. Nicholas Ouellette is a Professor of Civil and Environmental Engineering at Stanford University. He is broadly interested in the behavior of complex systems far from equilibrium, and in particular in the dynamical self-organization that is ubiquitous in such systems. Ouellette's current research interests include turbulence; the transport of inertial, anisotropic, and active particles by fluid flows; the strength and failure of granular materials; and collective behavior in insect swarms, bird flocks, and other animal groups. Ouellette graduated from Swarthmore College in 2002 with majors in Physics and Computer Science, earned his Ph.D. in Physics from Cornell University in 2006, and did postdoctoral research at the Max Planck Institute for Dynamics and Self-Organization and in the Physics Department at Haverford College. Before coming to Stanford, he spent seven years on the faculty in Mechanical Engineering and Materials Science at Yale University. He is a Fellow of the American Physical Society, and has won teaching awards at both Stanford and Yale.
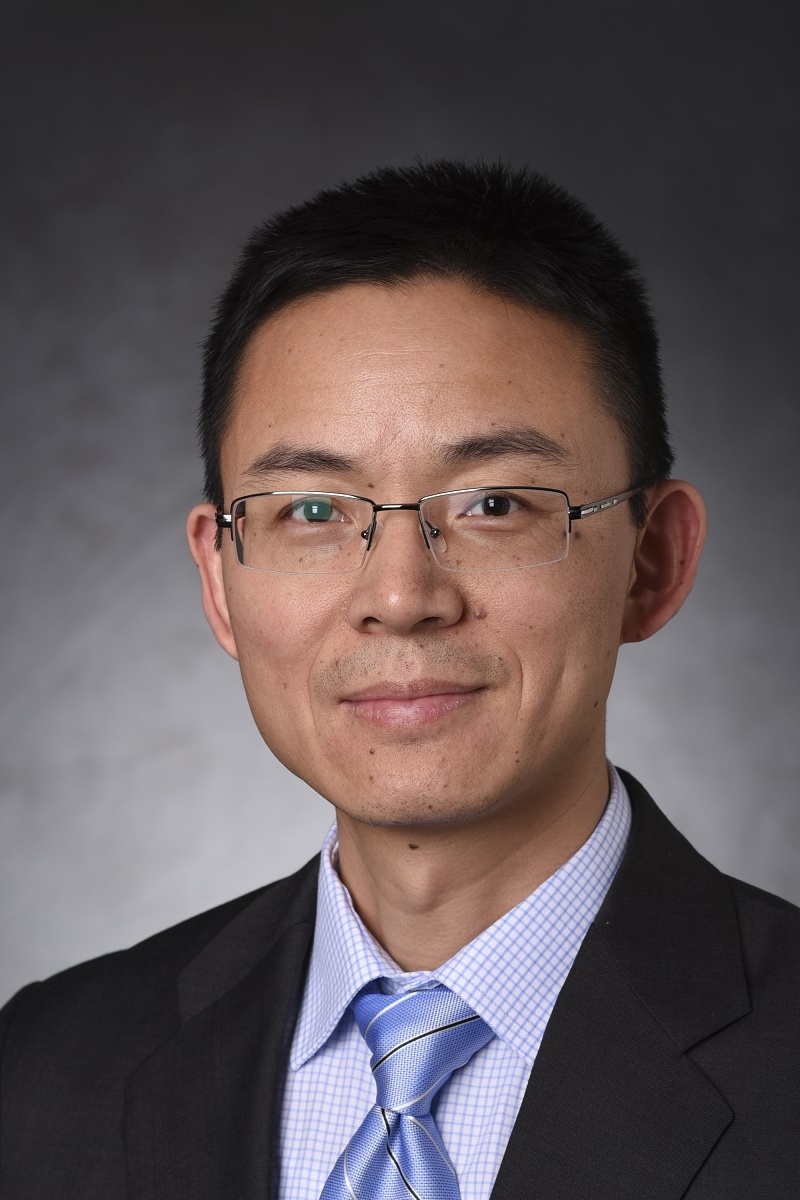
Xiaofeng Liu
Associate Professor, Department of Civil and Environmental Engineering
Pennsylvania State University
Physics-based and data-driven modeling in environmental fluid mechanics
February 2, 2023
Physics-based and data-driven models are two different, yet closely related, approaches for capturing processes in real world. This talk will provide a broad overview of both approaches and then showcase several examples in environmental fluid mechanics. Physics-based models (PBMs) solve governing equations using different numerical schemes. PBMs are predicated on the knowledge of physical processes and their mathematical representations (usually in the form of partial differential equations and constitutive relationships). I will show examples models for turbulent flow and sediment transport around structures/objects and fish passages. Data-driven models rely on the representativeness and quality of data to implicitly describe the physical processes. In high-dimensional space, data should be "big" enough to fully capture the input-output dynamics of a physical system. I will show examples of a deep-learning based surrogate for a 2D computational hydraulics model and its use for parameter inversion. Over time, the boundary between physics-based and data-driven models is blurred in the rapidly evolving arena of ML/AI. Hybrid approaches, such as physics-informed machine learning, have emerged. Some future research topics for environmental fluid mechanics will be discussed.
Biosketch
Dr. Xiaofeng Liu is an associate professor in the Department of Civil and Environmental Engineering at Penn State. He got his bachelor's degree in Hydraulic Engineering from Tsinghua University and a master's degree in Environmental Science from Peking University, China. He got his Ph.D. in Civil Engineering from the University of Illinois at Urbana-Champaign (UIUC) and a second master degree in Applied Mathematics in 2008. Dr. Liu's research interest includes computational fluid dynamics (CFD), sediment transport, and environmental fluid mechanics. His group specializes on the development and utilization of computational models for problems in environmental and water resource engineering. His research has been funded by NSF, U.S. Bureau of Reclamation, U.S. Army Corps of Engineers, USGS, DoD, DoE, DoT, and industry. Dr. Liu received the Harry West Teaching Award from Penn State and the State-of-the-Art of Civil Engineering Award from the American Society of Civil Engineers.
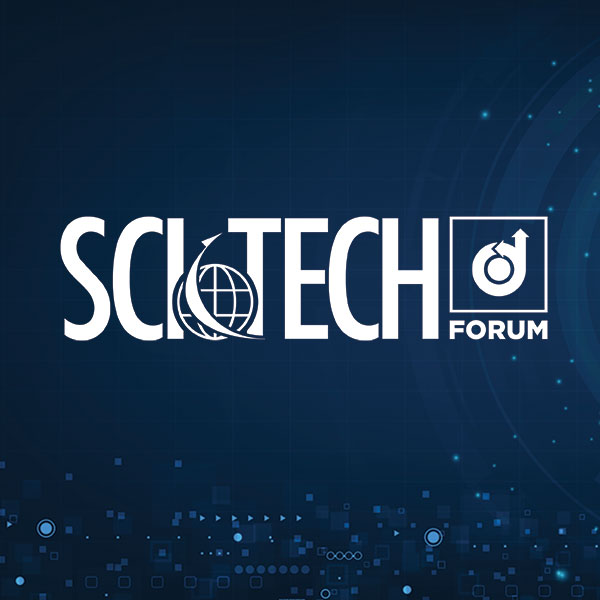
SciTech Blitz Talks
February 9, 2023
Relative impact of convective and radiative heat transfer in a backward facing step combustor
Jennifer Colborn, Department of Mechanical Engineering
Flow fields in gas turbine combustors have a wide variety of flow features near the combustor liner, including recirculation, shear layer separation and impingement, and boundary-layer development. As a result, modeling and predicting the heat transfer to the liner is challenging. The current work seeks to investigate the relative contributions of convective and radiative heat transfer to combustor liners using a backward-facing step combustor. Backward-facing step flows have many features relevant to gas turbines in a two-dimensional geometry, allowing for simpler modeling and easier instrumentation. Reynolds number, air temperature, and temperature of the combustor floor were varied to determine the impact of each parameter on the heat transfer through the different combustor zones: the recirculation, impingement, and recovery zones. Using a heat flux sensor and a radiometer, the total and radiative heat flux in these different zones was measured, respectively. Total heat flux was much higher than radiative heat flux, indicating the importance of convection heat transfer at these conditions. Increases in air and plate temperature increased both total and radiative heat transfer. Additionally, the highest heat flux was observed in the impingement zone and the lowest heat transfer was usually observed in the recirculation zone.
Implementation of two local correlation-based transition models in the OVERFLOW overset grid CFD solver
Samuel Gosin, Department of Aerospace Engineering
Menter's SST-based 1-equation transition model and an SA-based implementation of the Langtry–Menter γ–Reθ,t transition model has been implemented into NASA's OVERFLOW 2.3e CFD solver. An initial assessment of the implemented models has been carried out using benchmark 2D test cases involving flat plates and the NLF-0416 airfoil, focusing on bypass, Tollmien–Schlichting wave, and laminar separation bubble-induced transition scenarios.
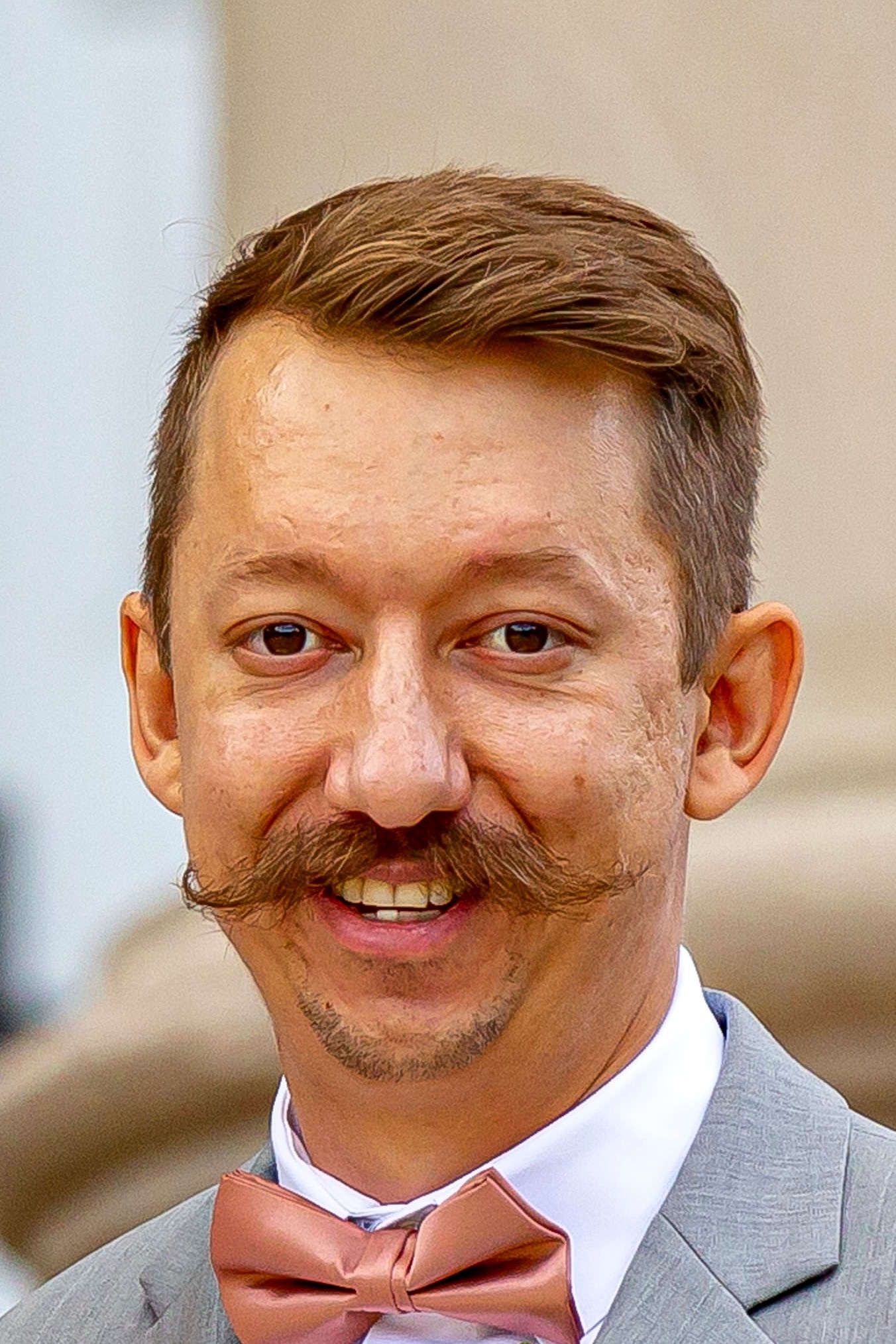
Matthew Berry
Hypersonic Aerodynamic Section Lead, Flight Sciences Department
Raytheon Missiles & Defense
Challenges in hypersonic design
February 16, 2023
Hypersonic flight has seen a significant increase in research/interest over the last decade. However, this flight regime poses unique design challenges. Raytheon Missile & Defense has been a major contributor in furthering hypersonic missile development. This talk will outline some of the challenges involved in the hypersonic air breathing and hypersonic boost glide missions.
Biosketch
Dr. Matthew Berry received his PhD in 2017 from Syracuse University focusing on flow control and reduced order modeling of complex supersonic nozzles. Following his doctorate, Matthew joined Raytheon Missiles & Defense in Tucson, AZ. Matthew has worked as an Aero Thermal Fluids Engineer, designing and modeling vehicles across a wide range of flight regimes, including underwater, subsonic, supersonic, and hypersonic systems. Matthew is currently the Hypersonic Aerodynamic Section Lead in the Flight Sciences Department.
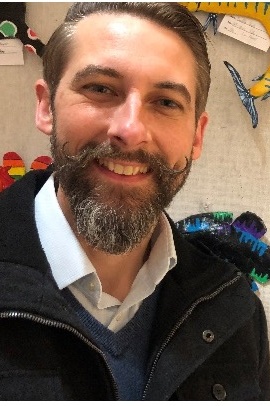
Todd Lowe
Professor, Kevin T. Crofton Department of Aerospace and Ocean Engineering
Virginia Tech
In 3D aerodynamics, is it the forest or the trees?
Local and large-scale aspects of turbulent flow over three-dimensional bodies
February 23, 2023
Three-dimensionality is the rule rather than the exception in air vehicle aerodynamics. In some cases, such as low Mach number boundary layer flow over high aspect ratio swept wings, the most important three-dimensional dynamics can often be explained by local effects. In other systems such as high-lift devices where the boundary layer may separate from the body, complex links among large-scale and local phenomena exist. In this talk, I will share the latest culmination of insights gained from the literature synthesized with new results being obtained from an on-going study of boundary layer separation over a three-dimensional hill. The contemporary study is termed Benchmark Validation Experiments for RANS/LES Investigations, or BeVERLI, while the associated geometry is termed the BeVERLI hill. This NASA-supported effort has the overarching goal of generating a new database of turbulence modeling benchmark data, but the test cases also present rich turbulent flow physics themselves worthy of deeper examination. Experimental results for the windward, attached boundary layer flows for the BeVERLI hill will be discussed regarding effects of boundary layer skewing, pressure gradient, and curvature. Additionally, mean flow symmetry breaking seen in both experiments and simulations will be discussed for a broad range of implications for flow physics, computational and experimental research approaches, and aerodynamic design.
For the last third of the talk, I will shift gears to provide a perspective on professional development, careers after graduate school, and share where some of the graduates from our team have gone in their careers.
Biosketch
Dr. Todd Lowe is a professor in the Kevin T. Crofton Department of Aerospace and Ocean Engineering at Virginia Tech. After receiving B.S., M.S., and Ph.D. degrees in aerospace engineering at Virginia Tech, he spent four years working in small business developing laser flow instrumentation, including work on a laser-Doppler velocimeter that would later be used in the NASA Juncture Flow Computational Fluid Dynamics Validation Experiment. In 2011, he rejoined Virginia Tech and now leads a research team focused on experimental aerodynamics and aeroacoustics, often addressing applications on propulsion inlets and exhausts. His fundamental contributions have provided insights for understanding turbulence transport and noise in turbulent shear flows, while parallel experimental technology research has advanced the state-of-the-art in nonintrusive flow diagnostics. He is co-inventor of four US utility patents, with four additional patents pending, and has co-authored more than 150 publications in the areas of advanced instrumentation, turbulent shear flow, supersonic jet noise, and turbulence modeling benchmark experiments. Among several leadership posts, he is the immediate past chair of the AIAA Aerodynamic Measurement Technology Technical Committee, a leader within the NATO STO Air Vehicle Technology activity AVT-349 Non-Equilibrium Turbulent Boundary Layers in High Reynolds Number Flow at Incompressible Conditions, the lead P.I. for a NASA NRA project on turbulence modeling benchmark experiments, and a co-P.I. for a newly awarded NASA ULI project on supersonic transport propulsion.
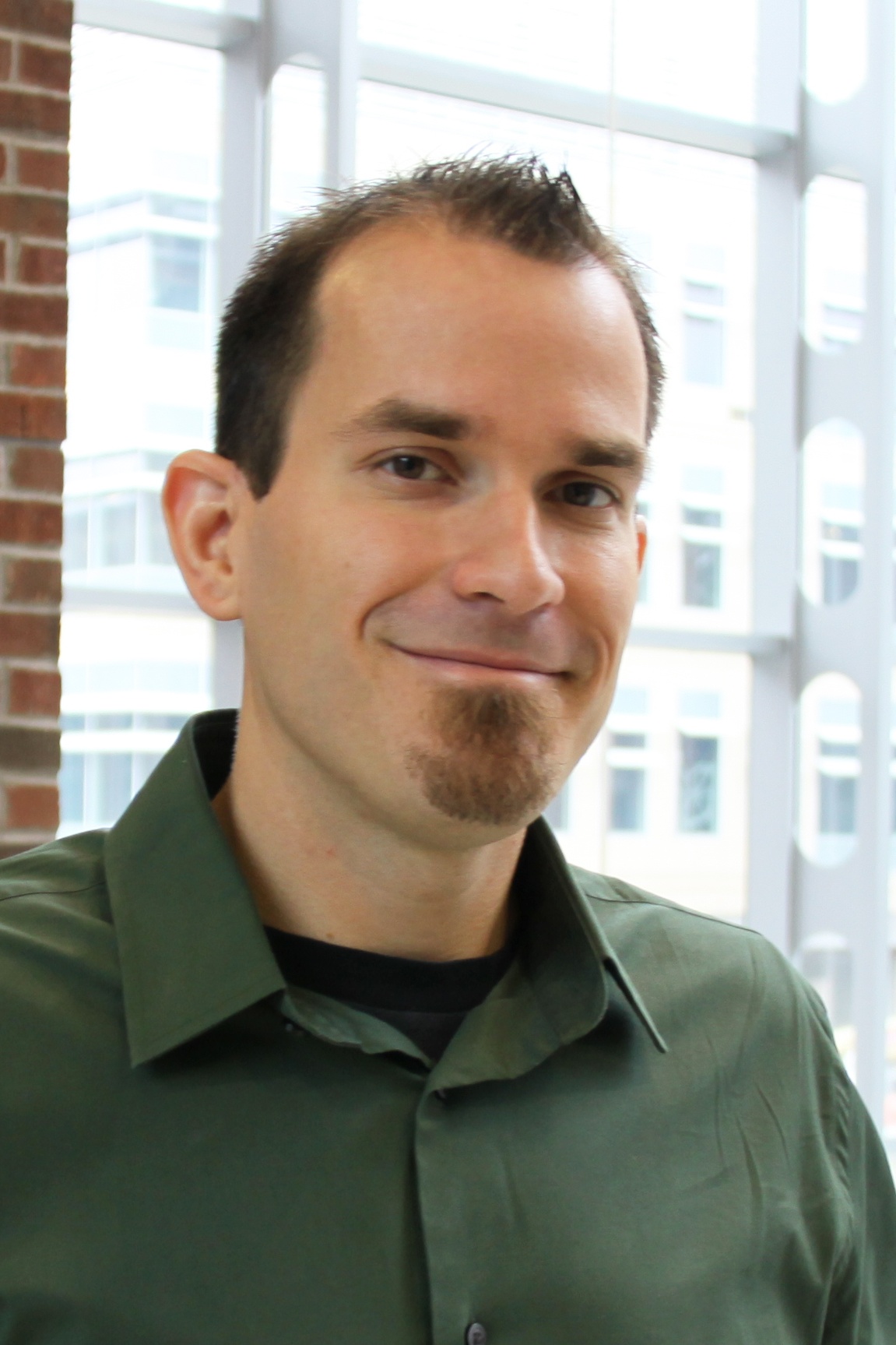
Jeffrey Sutton
Professor, Department of Mechanical and Aerospace Engineering
Ohio State University
High-resolution velocimetry for turbulent flows
March 2, 2023
Turbulent flows are ubiquitous in nature and engineering systems, thus necessitating an understanding of the basic physics governing their behavior. In experimental fluid dynamics research, quantitative velocity measurements are paramount for describing flows, their impact on nearby bodies, and the generation of aero/hydrodynamic forces that underpin power generation, ground transportation, and aerospace systems. For turbulent flows, experimental requirements are stringent due to their highly three-dimensional nature and broad range of length and time scales. For the last three decades, optical and laser-based measurement approaches have emerged as the primary investigative tools with particle image velocimetry (PIV) becoming the de facto method for determining fluid velocities. Traditional PIV (based on cross correlation processing) is well established but suffers from spatial resolution limitations which can lead to inaccuracies in high-gradient regions of turbulent flows and in calculating critical derivative quantities. Optical flow motion estimation (OFME) presents an alternative to traditional cross-correlation-based PIV and produces velocity fields that are dense (one vector per pixel) and more accurate. In this talk, I will discuss the development and application of one particular OFME approach, termed wavelet-based optical flow velocimetry (wOFV). wOFV provides the opportunity to increase measurement spatial resolution by more than a decade with significant increases in accuracy. Specific case studies using synthetic and experimental data will be presented that quantify the gains in resolution and accuracy using wOFV compared to traditional PIV. I will discuss recent extensions of planar, two-component wOFV to stereoscopic and tomographic implementations and finally discuss future directions and implementations into challenging environments such as hypersonic boundary layers.
Biosketch
Prof. Jeffrey A. Sutton is a Professor within the Department of Mechanical and Aerospace Engineering at Ohio State University and the director of the Turbulence and Combustion Research Laboratory. He received his Ph.D. in Aerospace Engineering from the University of Michigan in 2005 and was a National Research Council Postdoctoral Fellowship within the Chemistry Division of the Naval Research Laboratory in Washington, D.C. from 2005 to 2008. Subsequently, Prof. Sutton joined the faculty at Ohio State University in 2008. Prof. Sutton's current research interests include turbulent mixing and combustion, the development and application of advanced laser diagnostics, vaporization and gas-phase mixing in high-pressure turbulent sprays, auto-ignition dynamics, particle-laden flows, energetics, and high-speed propulsion. Prof. Sutton is an Associate Fellow of AIAA, Associate Editor of the Proceedings of the Combustion Institute, a member of the Editorial Board of Combustion and Flame, and author of more than 100 journal and conference publications. He is the recipient of the National Science Foundation CAREER award, the Air Force Office of Scientific Research Young Investigator Program award, the Ohio State University College of Engineering Lumley Research award, the Distinguished Paper award at the 31st International Symposium on Combustion, and two Best Paper awards from AIAA.
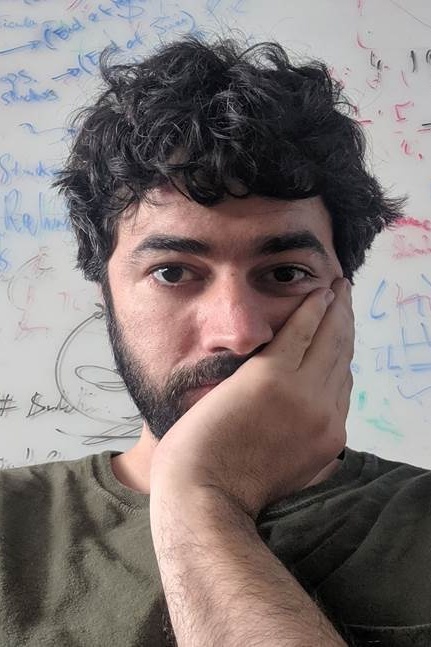
Christopher Crowley
Postdoctoral Fellow, Department of Mechanical Engineering
Johns Hopkins University
Experimental evidence that turbulent evolution can be approximated by recurrent flows
March 16, 2023
Despite a long and rich history of scientific investigation, fluid turbulence remains one of the most challenging problems in science and engineering. One of the key outstanding questions concerns the role of coherent structures that describe frequently observed patterns embedded in turbulence. It has been suggested, but not proven, that coherent structures correspond to unstable, recurrent solutions of the governing equations of fluid dynamics. In this talk, I will present the first experimental evidence that three-dimensional, weakly turbulent (spatiotemporally chaotic) flow tracks the spatial and temporal structure of multiple such solutions, episodically but repeatedly. These results provide compelling evidence that coherent structures, grounded in the governing equations, can be harnessed to predict how turbulent flows evolve.
Biosketch
Dr. Christopher Crowley is a postdoctoral fellow at Johns Hopkins University, where he works with Prof. Rui Ni studying vortex breakup in stratified flows such as those observed in the ocean. His main research interests are in dynamical systems modeling of complex phenomena such as turbulence and developing novel measurement techniques to provide more information about these systems. Chris started his scientific career at the National Institute of Standards and Technology (NIST) right after graduating from high school. Upon co-authoring his first paper, he matriculated at the University of Maryland (UMD), where he studied both physics and philosophy while continuing to work at NIST. By the time he graduated, Chris authored or coauthored 9 peer-reviewed publications and 2 NIST Special Publications. His research at NIST was focused on flow metrology, but he became increasingly interested in understanding turbulence deterministically. After graduating from UMD, Chris went on to the Georgia Institute of Technology (Georgia Tech), where he received his master's and PhD in physics, working with Profs. Mike Schatz and Roman Grigoriev on a dynamical systems description of turbulence. In addition to studying turbulence at Georgia Tech, Chris pursued numerous side projects, including developing a technique for simultaneously measuring spatiotemporally-resolved ion and voltage waves in cardiac tissue, studying how Hawk Moths fly, patenting a medical device, and starting a company.
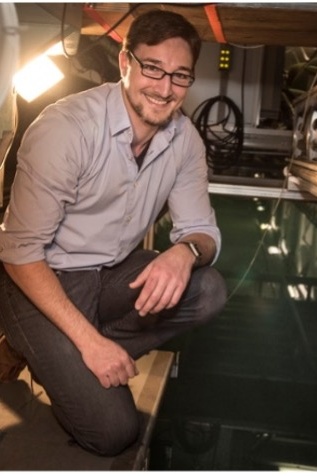
Casey Harwood
Assistant Professor, Department of Mechanical Engineering
University of Iowa
System identification of fluid–structure interactions with kinematic shape sensing
March 23, 2023
Fluid–structure interactions (FSIs) can drive radical changes in the response behavior and stability of immersed structures, with outcomes ranging from the catastrophic structural failure of civil structures to the beneficial delay of stalled flow in high-lift aerospace control surfaces. Despite the ubiquity and importance of FSI in marine settings, direct observation of compliant structures is difficult to achieve. This talk will describe recent advances in the sensor design and modeling methodologies being used to study complex fluid–structure interaction problems. A robust, low-cost kinematic shape sensor (KSS) has been designed to provide 3D shape reconstruction with precision near that of photogrammetric methods, but without the need for optical access. When paired with robust parameter estimation algorithms, such as stochastic subspace identification, the KSS affords extremely accurate resolution of mode shapes and modal parameters for structures excited by ambient flow conditions. A co-analysis framework is introduced, wherein output-only structural analysis is executed concurrently with spectral proper orthogonal decomposition of a secondary videographic dataset, providing insight into synchronous fluid modes accompanying or exciting structural vibration. This FSI co-analysis is used to demonstrate modal coalescence, cavitation-induced vibration, and vortex lock-in of a compliant lifting surface in multi-phase flow. Applications of these new analytical techniques will be discussed, including the spatial model realization of immersed structures and the use of targeted fluid-structure resonances to achieve flow control.
Biosketch
Dr. Casey Harwood is an Assistant Professor in Mechanical Engineering and an Assistant Research Engineer with IIHR—Hydroscience and Engineering. Dr. Harwood received his M.S.E. and Ph.D in Naval Architecture and Marine Engineering from the University of Michigan and his B.S., also in Naval Architecture and Marine Engineering, from the Webb Institute in New York. At the University of Iowa, Dr. Harwood leads the Marine Science and Technology (MaST) lab, which performs research in experimental marine hydrodynamics. His current areas of focus include fluid–structure interactions, model-scale testing of marine vehicles, amphibious vehicle design and control, and surf-zone hydrodynamics. He is the recent recipient of an NSF CAREER award to experimentally study fluid–structure interactions for improved modeling and active control of fluid-loaded structures.
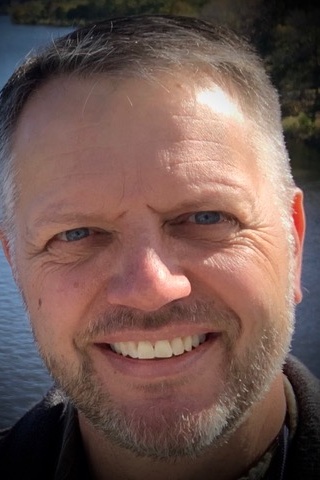
David Darmofal
Jerome C. Hunsaker Professor, Department of Aeronautics and Astronautics
Massachusetts Institute of Technology
Recent explorations of higher-order adaptive methods in fluid dynamics
March 30, 2023
In this talk, we will discuss some recent work in the application of higher-order and adaptive methods to fluid dynamics. First, we will consider the use of higher-order, adaptive finite element methods to sonic boom propagation. The computational cost to analyze and design the next generation of low boom aircraft is significant as a direct result of the increasingly tighter control required on sonic boom noise generation. We will provide a general overview of the sonic boom modeling process and then focus on the development of an adaptive finite element method to solve the boom propagation problem. Results demonstrate that the new adaptive method can efficiently and reliably produce high accuracy solutions to the boom propagation problem.
Second, we will consider the impact of higher-order methods in the simulation of chaotic problems, such as Large Eddy and Direct Numerical Simulations. We develop a simple model for discretization and statistical errors, including effects of start-up errors as well as parallel ensemble averaging. Then, we analyze the model to show how the potential benefits of higher-order discretization are significantly constrained by statistical errors.
Biosketch
Dr. David Darmofal is the Jerome C. Hunsaker Professor of Aeronautics and Astronautics at MIT and a member of the MIT Aerospace Computational Design Laboratory (ACDL) and the MIT Center for Computational Science & Engineering (CCSE). His principal areas of interest are computational methods for partial differential equations, especially fluid dynamics; and engineering education innovation. Darmofal earned his BS in Aerospace Engineering from the University of Michigan (1989), and SM (1991) and PhD (1993) in Aeronautics and Astronautics from MIT. From 1995–1998, he was an assistant professor in the Department of Aerospace Engineering at Texas A&M University. He joined MIT in 1998, became a full professor there in 2010, and has served as the Department of Aeronautics and Astronautics associate and interim chair.
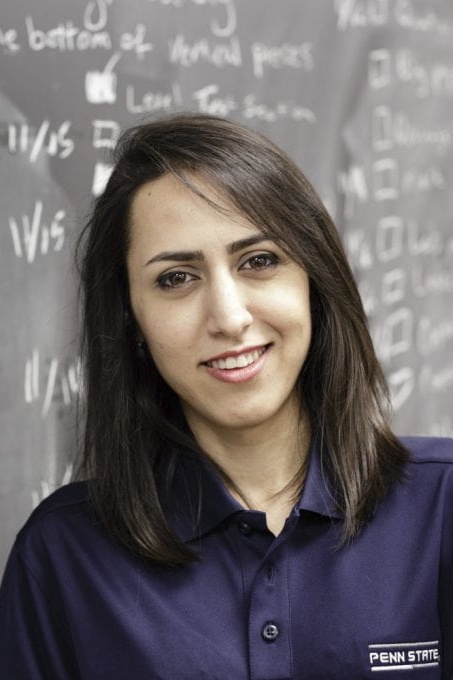
Azar Panah
Associate Professor, Division of Engineering, Business and Computing
Penn State Berks
Aerodynamics of flapping wings in gusty environments
March 31, 2023
Birds and insects can stabilize themselves in gusty environments with their flapping wings, surpassing what fixed-wing aerial vehicles can do in similar conditions. We hypothesized that the flapping movements of a wing can indeed suppress the effects of incoming gust structures by studying both the fluid flow surrounding the wing and the wing's aerodynamic loading. Particle Image Velocimetry (PIV) was used to observe the flow around the wing, and a force/torque transducer was used to measure the loads acting on it. With increasing Strouhal number, the circulation and the forces increased in magnitude, but the increase in the spread of both quantities, due to the gusts, lowered. This suggests better predictability of the flow and forces for plunging wings at higher St, which could be exploited for better stabilization against gusts.
Gallery of Fluid Motion and Flow Visualization Instruction
The Gallery of Fluid Motion is intended to be a visual record of the
aesthetic and science of contemporary fluid mechanics, to be shared
both with fellow researchers and the general public. An experimental
course on flow visualization is offered to PSU students as a General
Education course (PHOTO 321N). This course is part of the new GenEd
integrative studies requirement intended to make integration between
two knowledge domains: Art and Physics of Fluids. Students explore a
variety of aesthetic issues in the form of practical and creative
assignments. The course objectives are "integrative thinking",
"creative thinking", and "effective communication". Student projects
are presented as a small Gallery of Fluid Motion at Berks. Best
samples of student works are entered to the Annual Gallery of Fluid
Motion competition at DFD which enables students to network with the
scientist in the field. This course has proven to be very successful
in attracting all students in both engineering and non-engineering
majors.
Biosketch
Dr. Azar Panah is an Associate Professor in Mechanical Engineering at Penn State Berks. She completed her Ph.D. thesis at the University of Iowa on flow structures of flapping wings to understand how birds fly and how we as engineers can design a robot to fly like birds more efficiently. She loves fluid mechanics and is fascinated by the mechanics of flying birds. Her research vision is to understand natural locomotion in fluids such as air and water, and if possible to design novel unmanned vehicles. She is always game to investigate an interesting question in fluid mechanics, especially if she can recruit her undergraduate students to sleuth around and wonder with her. She also teaches the science and photography of Flow Visualization to make the physics of fluid flows (gases, liquids) visible. In 2021, Dr. Panah became the Coordinator of Gallery of Fluid Motion at the American Physical Society’s Division of Fluid Dynamics (APS DFD), and it is an honor for her to serve the community in this capacity.
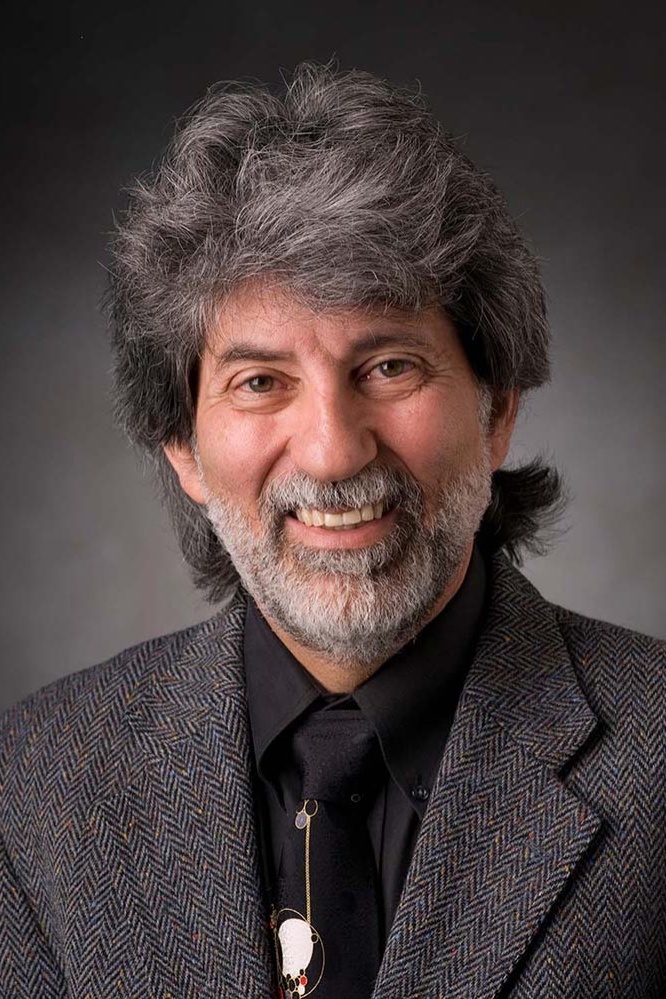
James Brasseur
Research Professor, Ann & H.J. Smead Department of Aerospace Engineering Sciences
University of Colorado Boulder
Impacts of atmospheric turbulence on wind turbine rotor aerodynamics and drivetrain main bearing function
April 6, 2023
This discussion will integrate three series of research studies into the nonsteady forces and moments that are generated at the rotor hub of utility scale wind turbines by the continual passage through the wind turbine rotor of the energy-dominant turbulence eddies that are embedded within the daytime atmospheric boundary layer (ABL). One series, developed at Penn State, applied large-eddy simulation (LES) of a wind turbine within the ABL to identify three characteristic time scales in the aerodynamic response to the passage of atmospheric turbulence eddies through the wind turbine rotor at the minute, second and sub-second time scales. These temporal scales are confirmed with analysis at Penn State of data from a GE field study. The third study was developed by comparing LES results with analysis at the University of Colorado Boulder (UCB) of field data obtained from the NREL/GE 1.5 MW wind turbine at the National Wind Technology Center, a site to the east of the Front Range of the Rocky Mountains. The NREL wind turbine responds to mountain-generated turbulence eddies embedded within westerly winds and confirms a key result from the LES-based study—that the continual passage of the energy-containing atmospheric eddies creates time-variations in the axial moment on the main shaft (torque and power) that are fundamentally different from the non-axial moment components that directly force the main bearing. The LES explains the turbulence-rotor interactions underlying these undamentally different responses. The implication is that that methods to suppress the potentially deleterious impacts of atmospheric turbulence on main bearing failure (the numerator in the levelized cost of energy (LCOE)) must differ fundamentally from methods designed to suppress turbulence-induced time variations in power (the denominator in LCOE). Current research at UCB integrates aerodynamics-based models with models of the main bearing to determine the extent to which atmospheric turbulence increases main bearing failure and LCOE.
Biosketch
Dr. James Brasseur is currently Research Professor of Aerospace Engineering Sciences at the University of Colorado Boulder after 27 years as Professor of Mechanical Engineering, Biomedical Engineering and Mathematics at the Pennsylvania State University, where he retains Emeritus Professor status. Dr. Brasseur is a fluid dynamist with extensive expertise in turbulence physics, turbulent flows, and large-eddy simulation, including atmospheric and reacting turbulent flows. Dr. Brasseur has separate expertise in the mechanics and physiology of the gastro-intestinal tract, including pharmaceutical release, transport and absorption. Dr. Brasseur completed his Ph.D. in 1979 from the Department of Aeronautics and Astronautics at Stanford University and completed postdoctoral positions at NASA-Ames (CFD), University of Southampton (aerodynamics) and Johns Hopkins University (turbulence). He has been visiting professor/scientist at a number of institutions and has served on governing boards of the American Physical Society (APS) and the APS Division of Fluid Dynamics, was past president of a medical society, and was founding Chair of the APS Topical Group on the Physics of Climate. Dr. Brasseur is a member of the Johns Hopkins Society of Scholars and is Fellow of the American Physical Society.
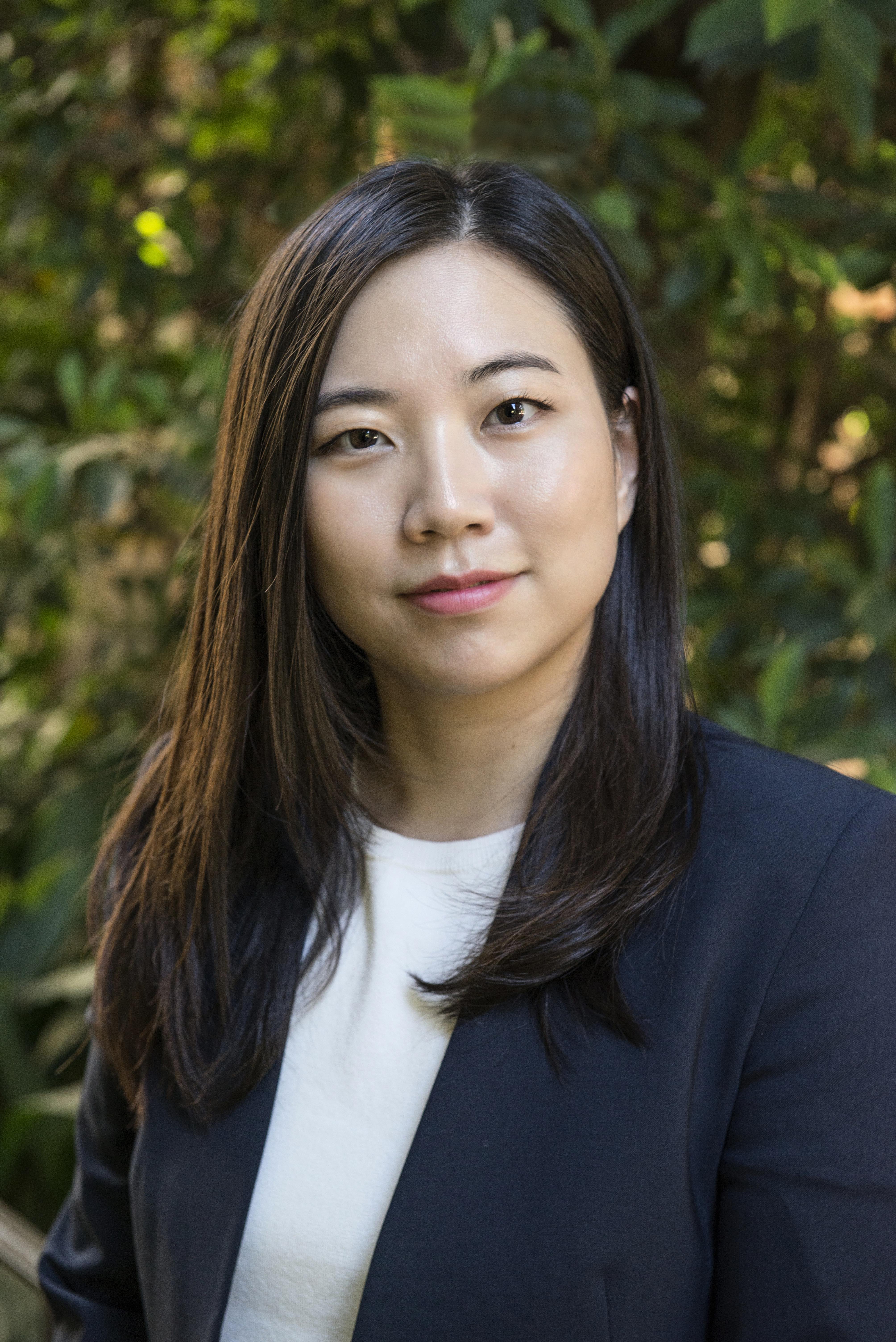
Jane Bae
Assistant Professor, Graduate Aerospace Laboratories (GALCIT)
California Institute of Technology
Nonlinear mechanisms of wall-bounded turbulent flow
April 13, 2023
In this talk, I will discuss the nonlinear interaction in the self-sustaining process of wall-bounded turbulence. Resolvent analysis is used to identify the principal forcing (nonlinear) mode which produces the maximum amplification in direct numerical simulations of the minimal channel for the buffer layer. The identified mode is then removed from the nonlinear term of the Navier–Stokes equations at each time step from a direct numerical simulation of a minimal channel. The results show that the removal of the principal forcing mode is able to inhibit turbulence in the buffer layer, while the removal of subsequent modes only marginally affects the flow. Analysis of the dyadic interactions in the nonlinear term shows that contributions toward the principal forcing mode come from a limited number of wavenumber interactions. Using conditional averaging, the flow structures that are responsible for generating the principal forcing mode, and thus the nonlinear interaction to self-sustain turbulence, are identified to be spanwise rolls interacting with meandering streaks. Finally, I will introduce an extension to resolvent analysis for non-stationary flow.
Biosketch
Dr. Jane Bae is an Assistant Professor of Aerospace at the Graduate Aerospace Laboratories at Caltech. She received her Ph.D. in Computational and Mathematical Engineering from Stanford University in 2018. She was a postdoctoral fellow in the Graduate Aerospace Laboratories at Caltech and the Institute for Applied Computational Science at Harvard University before joining the Caltech faculty. Her main research focuses on computational fluid mechanics with emphasis on modeling and control of wall-bounded turbulence.
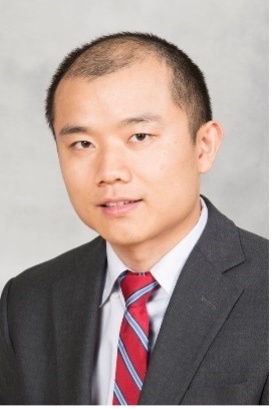
Jifu Tan
Assistant Professor, Department of Mechanical Engineering
Northern Illinois University
Multiphysics modeling and parallel computing in flows with cell suspensions
April 20, 2023
Fluid flows containing solid particles are common in engineering and biology, e.g., complex flow with cellular suspensions. The dynamic behavior in such phenomena is complex and poorly understood due to interactions between individual particles as well as interactions between the particles and the surrounding fluid and bounding walls. Moreover, the presence of highly deformable particles, such as blood cells, vesicles and polymers, make it particularly challenging to accurately describe the dynamics in such systems. In this presentation, a massively parallel Multiphysics simulation platform developed on popular open-source code will be introduced to simulate such complex flows. The fluid flow was solved by the Lattice Boltzmann method (LBM), while the solid deformation and diffusion were simulated by particle-based solver. The coupling was achieved through the immersed boundary method (IBM) so that different physics can exchange information with each other. The developed simulation framework was validated with various analytical solutions and experiments and shown to scale almost linearly over thousands of processors. Applications are given in drug delivery, cancer cell detection in microfluidics, and blood flow in a retina vascular network, demonstrating an efficient way of simulating coupled multi-physics problems.
Biosketch
Dr. Jifu Tan currently is an assistant professor in Mechanical Engineering at Northern Illinois University. Before that, he was a postdoctoral associate in the Department of Chemical and Biomolecular Engineering at the University of Pennsylvania. He obtained his Ph.D. and M.S. in Mechanical Engineering from Lehigh University in 2015 and 2012, respectively. He received his B.S. degree in Civil Engineering from Beijing Jiaotong University in 2007. His primary research interest is fluid structure interaction and its application in engineering and medicine, such as nanoparticle delivery, blood flow modeling, thrombosis and bleeding simulation, microfluidic device design for cell separation. He is also interested in high performance computing, multiscale modeling, and data driven models. He is actively engaged in developing open-source code for scientific research.
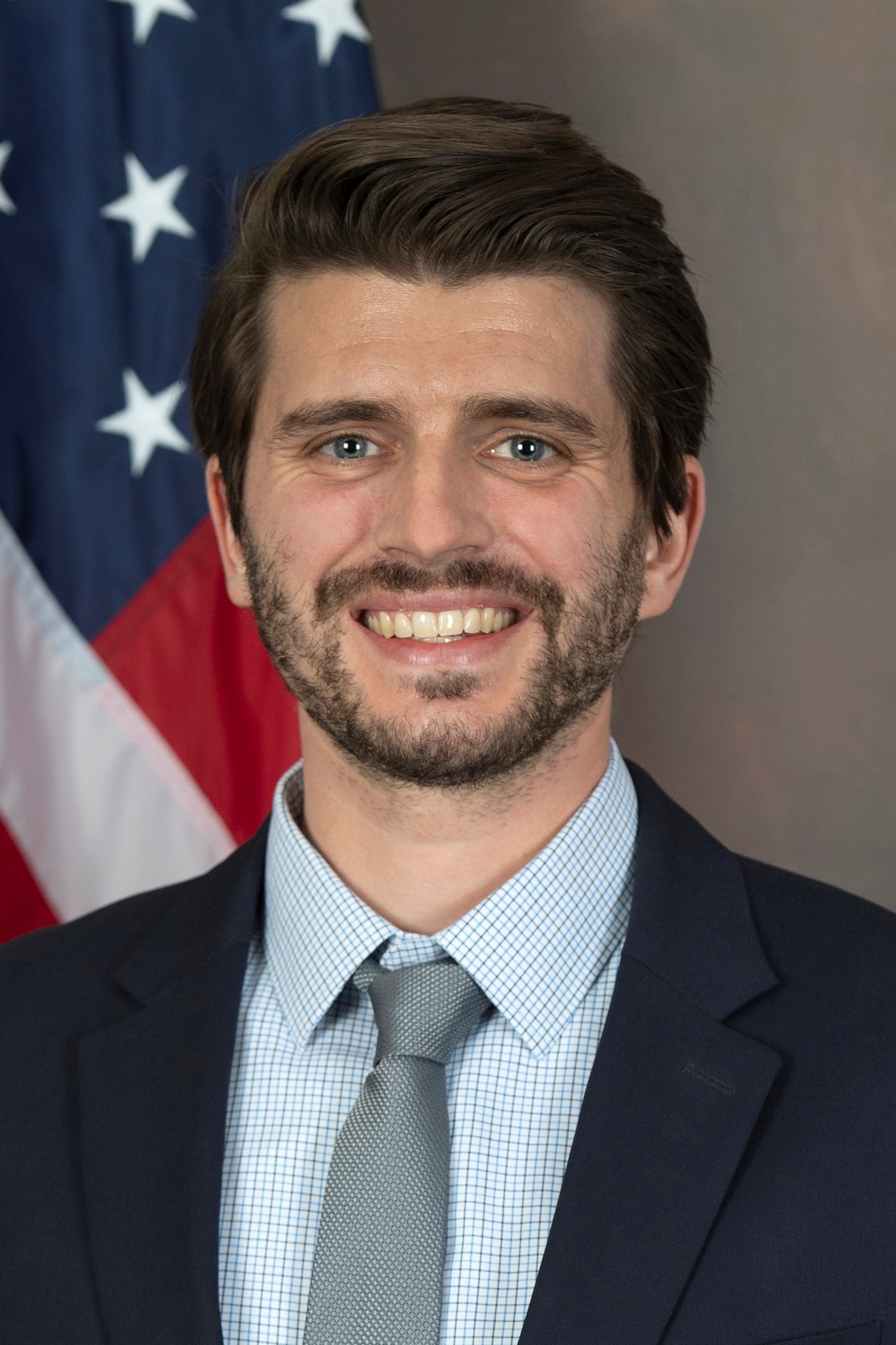
Andrew Banko
Assistant Professor, Department of Civil and Mechanical Engineering
United States Military Academy, West Point
New approaches to the measurement and modeling of passive scalar transport in flows with strong three-dimensionality
April 20, 2023
Turbulent mixing in geometrically complex systems underpins many fundamental challenges in the development of sustainable environmental and advanced energy solutions. Flows bridging scales from the urban canopy to compact heat exchangers contain strong three-dimensionality which pushes the limits of conventional gradient-diffusion scalar transport models. This is because standard Reynolds-Averaged Navier–Stokes (RANS) models were developed, for good reason, using simplified building-block flows with limited measurements. In this talk, I'll present two parallel lines of effort to improve scalar transport models that provide and utilize fully three-dimensional data in complex flows. First, magnetic resonance imaging techniques to measure velocity and scalar concentration fields without the need for optical access are discussed, beginning with fundamental principles and concluding with insights gained on topological dispersion in urban canopy flows. Second, a physics-constrained neural network approach to improve turbulent scalar flux models is presented. Emphasis is placed on identifying and explaining model extrapolation, and how flow dimensionality within the training database plays a critical role. Finally, I provide an outlook on new opportunities to combine advanced diagnostics with physics-constrained data-driven modeling.
Biosketch
Dr. Andrew Banko is an assistant professor in the Department Civil and Mechanical Engineering at the United States Military Academy (West Point). He earned his B.S. in Mechanical Engineering from the University of Illinois at Urbana-Champaign, and his M.S. and Ph.D. in Mechanical Engineering from Stanford University. Prior to joining West Point, he was a post-doctoral fellow at Stanford's Center for Turbulence Research. His research investigates turbulent mixing in applications spanning the environment, energy, and human health. He is particularly interested in how microscale turbulent dynamics interact with complex geometry or multiphase components to impact large-scale flow properties such as heat and mass transfer rates. Current projects are focused on particle-based solar receivers, gas turbine cooling systems, aerosol transport in indoor spaces, and the dispersion of contaminants within urban environments. At West Point, he conducts research with a talented team of undergraduate students, with teaching and mentorship at the core of each project.